Research papers
← vista completaPublished on April 15, 2019 | http://doi.org/10.5867/medwave.2019.03.7611
Reliability of two gait speed tests of different timed phases and equal non-timed phases in community-dwelling older persons
Fiabilidad de dos pruebas de velocidad de marcha de diferentes fases cronometradas e iguales fases no cronometradas en personas mayores que viven en la comunidad
Abstract
Background Gait speed is a fast, low cost and accurate measurement for evaluating older persons’ functional ability, both health and with comorbidities. Previous studies have evaluated gait speed measured over courses of varying distances, but the non-timed phases are not measured uniformly. It is unknown if this affects the results of the test.
Objective This study aims to evaluate the reliability of the running speed test of two different timed phases compared to the same nontimed phases.
Methods We conducted a descriptive reliability study, with an observational and cross-sectional analytical design. One hundred thirty-six older persons were included. Two gait speed tests were taken, one of 4 and 10 meters, and 2 meters for the acceleration/deceleration phase for both tests. The average of two attempts was obtained as a final measure of each test. The intraclass correlation coefficient was used to express the results (ICC) with a fixed effects model and the Bland and Altman method (confidence interval of 95%), complemented with the standard error of the mean and minimal detectable change with 95% confidence values (MDC95).
Results The results indicate an excellent level of agreement between the attempts of the tests of 4- and 10-m (ICC = 0.959 and 0.976, respectively), as well as between the average of the two tests (ICC = 0.867). The agreement was slightly better between the two attempts in the 10-meter test. The number of attempts does not affect the results of gait speed. Further analysis is required to conclude the same regarding the distance of the test (4 and 10 meters). The difference in the results of the Bland Altman analysis for the average of the 4 and 10-meter tests was 1.5945 m/s (95% confidence interval: 0.9759 to 2.2130 m/s), which is too wide and higher than the MDC95 value.
Conclusions The ICC value was excellent in all cases, and the number of attempts does not affect the results of gait speed. However, further analysis is required to conclude the same regarding the distance of the test. There is an insufficient agreement between the two tests to allow them to be used interchangeably in populations with the characteristics of this study.
Key ideas
|
Introduction
Functionality is fundamental in the evaluation of older persons and may increase the value of the traditional clinical exam [1]. In the literature, different methods have been described to measure it, but one of them has stood out and caused great interest in the scientific society, the evaluation of the gait speed [2]. Within the functional evaluation of older persons, gait speed has even been cataloged as the “sixth vital sign” of those advanced in age as it reflects both functional and physiological changes [3].
Gait independence is of great relevance to older persons as their locomotive capabilities become more likely to diminish, starting a degenerative process of the physical, psychological and social state of functionality [4],[5]. Gait speed has proven to be a reliable measure for evaluating the older persons' functional capacity, also providing predictive information about health-related issues, such as the risk of falling, the risk of suffering fractures and the risk of being hospitalized or even rehospitalized [6],[7]. In addition, a predictive factor in cardiovascular health and mortality is also considered [4],[8], making it comparable to other well-known functionality performance measures, such as the 6-minute walk test [5],[9],[10],[11]. In the study conducted by Busch et al. (2015)[1], a relationship between gait speed and some modifiable factors, such as a decline of daily activities, physical inactivity, and cardiovascular illnesses, was evidenced.
Caetano et al. (2017)[12] reported that lower gait speeds could serve as a predictive factor in detecting a high risk of falling. Decreased executive function, increased concern for falling and weaker quadriceps function also contributed to this relationship. Additionaly, Aranda Garcia et al. (2015)[13] noted that the best maximum gait speed predictor is the variable of leg strength, followed by age and the level of physical activity as general variables.
Gait speed evaluation is a procedure that is easy to conduct, requires little time, has low related costs and doesn’t require a complex clinical scenario other than the walking platform itself, with the measures suggested in the literature and according to the available reality. [14]. The basic supplies needed to run the test are chronometer and a flat surface to walk on, of a distance preferred and previously determined by the evaluator [15]. When it comes to conducting the test, some authors agree that the participant should walk 2 or more times so that an average can be calculated to obtain a more reliable speed gait result [16],[17],[18]. In terms of the most appropriate way to measure the time it takes the participant to move between the two specific places, Peters et al. (2013)[19] showed that there were no statistically significant differences between the use of a manual chronometer and a wireless measuring system. Indeed, they declare that stopwatches are often used in both clinical and research settings to record walking time in the calculations of gait speed and are a more accessible instrument that automatic timers, they are simple, but few clinical settings have such devices.
There are many different specifications in terms of a testing protocol; for example, gait speed can be measured for both self-selected and “fast” walking speeds, using a static or dynamic start, and over a variety of distances [20]. In the literature, most studies chose distances between 4 and 10 m, though some have used longer distances [4]. For practical reasons, there may not be an ideal test protocol. However, the ease of altering walking speed by clinically significant amounts simply by changing the protocol demonstrates the need for consistency in the test protocol [8]. Certainly, the physical conditions and real-life scenarios that participants face in their daily lives differ widely; for example, gait speed assessment using a walking start may not be feasible in all clinical settings, particularly those that have limited space and would not allow non-timed phases (acceleration/deceleration), like is some home cares and hospital rooms situation [8].
Consequently, it is necessary to know if this test is applicable regardless of the inclusion of the timed and untimed distance, as well as the differences of this between different studies. Undoubtedly, this information would allow clinicians to more easily choose the most appropriate protocols in terms of available physical space. It is important that both clinicians and researchers are aware of the significant impact of altering the aspects of the protocol test on the measured walking speed. To our knowledge, no head-to-head comparison between static and dynamic starting distance protocols has ever been performed among the various methodologies previously used to assess the gait speed. Therefore, this study aimed to evaluate the reliability of the running speed test of two different timed phases and the same nontimed phases.
Methods
Participants
We conducted a reliability study of descriptive scope, with an observational and cross-sectional analytical design, including 136 older persons belonging to different community groups from the Concepción province (Bio-Bío Region, Chile), participated as a non-probabilistic sample for convenience, between October and November of 2017. Researchers formally contacted these subjects through personal interviews. Each participant signed a letter of consent approved by the Ethics committee of the Universidad de las Américas (UA03). The study was approved by the institutional ethics committee of the Universidad de las Américas.
The inclusion criteria of the study were: male and female individuals, aged between 65 and 90 years old, able to walk with or without aids of any kind, totally independent in their activity of daily living (with score >80 on the Barthel Index), and capable of understanding and following instructions (with score ≥14 in the abbreviated Mini-Mental State Examination) and participated actively in their community groups. Those who presented any musculoskeletal injuries that impeded walking or regularly consumed any depressants of the central nervous system or depressants of labyrinthine-vestibular function were excluded from this study, these antecedents were previously consulted in an interview and registered in a clinical record. Originally 153 older people were invited to participate, but only 136 performed and completed the gait speed tests. The 17 excluded subjects were excluded from the analysis by incomplete data screened at the interview stage.
Procedure
The subjects could walk with or without walking aids, but there were no cases that required it. The subjects were instructed not to exercise before the test beyond their normal exercise activities and to wear comfortable footwear.
Each participant took two gait speed tests of different distances but with the same instructions. The first test considered a distance of 14 meters in total, consisting of 2 m of acceleration, followed by 10 m of walking measured with a chronometer, and finally 2 m of deceleration. The second test of 8 m total began with 2 m of acceleration, followed by 4 m of measured walking time, and then 2 m of deceleration (Figure 1). The order of administration of the two different protocols was randomly varied among participants. Both tests start with a verbal signal once the participant is in a standing position and both feet are behind the starting line. Each participant must walk as fast as possible, without running or stopping towards the finish line. Participants performed each test twice, with a one-minute break between each attempt [16]. No verbal or motivational stimuli were given during the tests. Evaluations were conducted in a standardized instruction way by one researcher who performed all the measurements of the chronometer to avoid the introduction of variability among the evaluators; expertise and attention are required when managing the time measurement system [21].
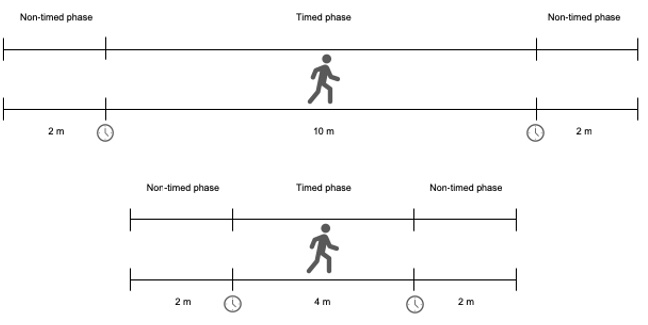
The gait speed was calculated by dividing the number of meters of measured walking distance by the time it took the participant to complete the test, resulting in a variable expressed in meters per second (m/s).
Data analysis
We used descriptive and inferential analysis. The Kolmogorov-Smirnov test was used to test for normality of data. To establish the relationship between 2 different tests that measure the same variable, the intraclass correlation coefficient (ICC) method was used with a fixed effect model. The ICC values were interpreted using the benchmarks suggested by Menz et al. (2004)[22]; when more than 0.75 excellent reliability; 0.40 to 0.75 fair to good reliability; and less than 0.40 poor reliability. The Bland and Altman method with a confidence interval of 95% in order also was used to evaluate the agreements between the first and second attempts for each gait speed test and the average between the 4 m and 10 m tests. The Bland-Altman technique allows visually assess the agreement between 4- and 10-m gait speed assessments. The resulting plot shows the size and range of the measurement differences and their distribution around the mean. The 95% confidence intervals provide an indication of how far apart measurements by the two gait speed tests are likely to be for most individuals [23]. To quantify the amount of change in gait speed that should be considered as excess measurement error and variability, the minimal detectable change (MDC) with 95% confidence was calculated using the formula: MDC95 = 1.96 X SEM X √2, where SEM is the standard measurement error. The SEM was determined by the formula [SD X √ (1 -r)], where r is the reliability coefficient (in this case, the ICC) and standard deviation of the test difference scores [24]. The analyses were conducted using IBM Statistic SPSS 22.0 software.
Results
One hundred and thirty-six independent older persons in activities of daily living were recruited from the community, ranging in age from 65 to 87 years old, with an average age of 72.8 (standard deviation 5.9) years, 75.7% were female, and 24.3% were male (Table 1). The descriptive results of the 4- and 10-meter gait speed tests are presented separately by attempts in the first and second columns of Table 2. The descriptive results of the mean of the two measurements for each test are presented in Table 3. The Kolmogorov Smirnov showed that gait speed measurements were normally distributed.
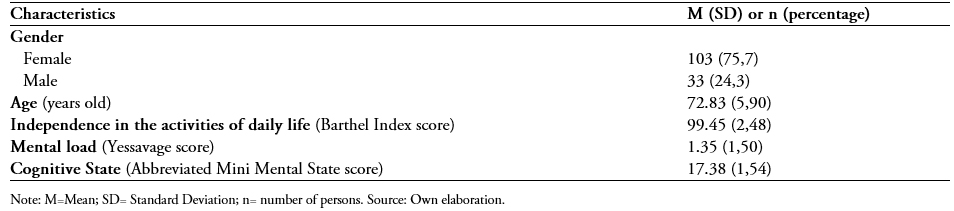


The ICC showed a high level of agreement between the first and second attempts in both the 4- and 10-m tests (ICC = 0.959 and ICC = 0.976, respectively) with SEM values between 0.053 and 0.067 m/s and MDC95 values between 0.146 and 0.185 m/s (Table 2). In Figure 2, the Bland and Altman plot shows that the average between the first and second attempt in the 4-m test was 0.0017 m/s (95% confidence interval: -0.2344 to 0.2830 m/s). In Figure 3, the average of both attempts in the 10-m test was 0.0017 m/s (95% confidence interval: -0.2090 to 0.2056 m/s). Whereas no obvious relationship between the difference and the mean was observed for any two attempts of 4- and 10- m gait speed assessment, a similar mean difference and 95% limit of agreement noted for both attempts, showing a slightly better level of agreement between the two attempts in the 10 m test.
Finally, the ICC between the average of 4- and the 10-meter test was excellent (ICC = 0.867), with 95% limits of agreement ranges from -0.813 to 0.905. The SEM value was 0.082 m/s and the MDC95 value was 0.227 m/s (Table 3). Figure 4 shows the mean of the differences between the average of 4 and 10-m tests, with a high value of the mean difference between the tests of 1.5945 m/s (95% confidence interval: 0.9759 to 2.2130 m/s).
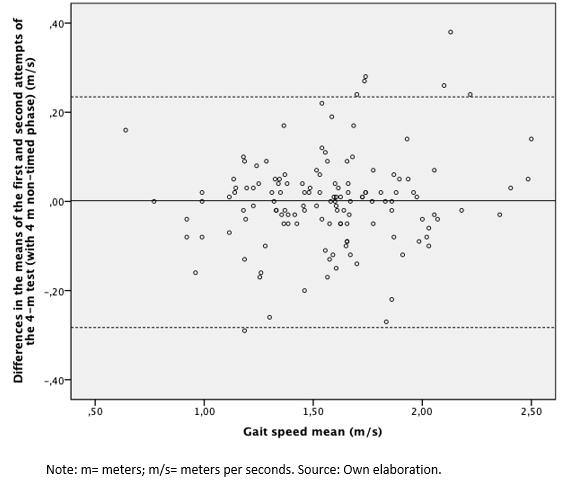
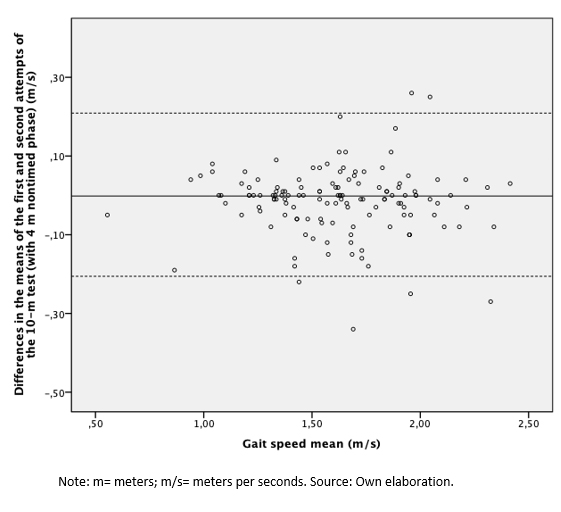
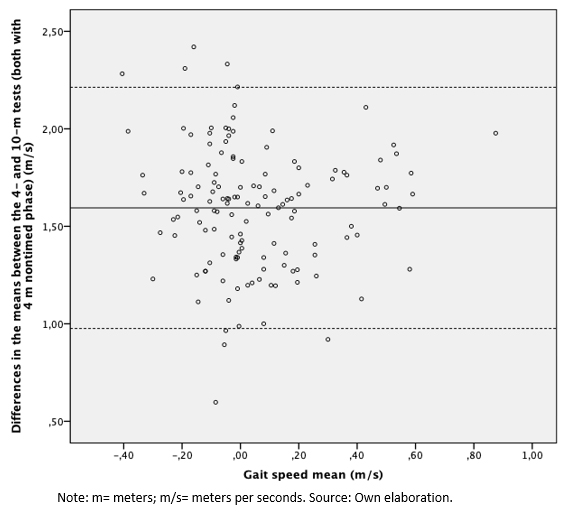
Discussion
The obtained results indicate an excellent level of agreement between the attempts of the tests of 4- and 10- m, as well as between the tests in independent dwelling-community older persons. The ICC was 0.959 and 0.976 for 4- and 10-m tests, respectively. However, Bland Altman's analysis of the 4-m test showed a small range between the limits of 95% agreement, where at higher average speed, there is a tendency to obtain greater differences in the speed calculated for the first and second attempt of the test. In any case, this interpretation can be subjective if small differences in the SEM values are analyzed because they indicate a clinically acceptable degree of agreement such that the intent number would not significantly affect the interpretation of the walking speed results. According to previous studies, the ICC values for similar objective groups varied between 0.79 and 0.98 for the gait speed in a test of the same distance [25],[26],[27]. In these studies, the method used to obtain the speed in meters per second was similar, but again and as Graham (2008)[28] also mentioned, there is no detailed report describing rhythm instructions, verbal stimulus or specific timing procedures. Although in our study the reliability of both gait speed tests is excellent, the 4-m test does exhibit a slightly down agreement compared to the 10- m test. When observing the difference in the results of the Bland Altman analysis for the average of the 4- and 10- m tests, this is too big and higher than the MDC95 value. Therefore, they couldn't be used interchangeably in this population.
As Varela et al. (2009)[2] stated, the ease of measuring gait speed and its usefulness in identifying people with a higher chance of developing certain harmful conditions, justifies its use in clinical practice and could lead to the development of other studies that could generate local registers of their region. The study conducted by Inzitari et al. (2016) is emphatic about the fact that the gait speed test fulfills the fundamental requirements to be considered an effective tool for sifting through different health-related conditions found in older persons but has yet to be present in clinical practice [29]. In contrast, the gait speed test has the benefit of being fast, safe, low cost and low risk. Regarding this last point, Andersson et al. (2011) say that any healthy older adult could perform the test as the changes in cardiac frequency, dyspnea, oxygen level, and effort are relatively low [30]. This was also confirmed by Bongers et al. (2015), whose study concluded that the gait speed test could be a safe and reliable tool with the potential to improve balance, mobility and diminish the risk of falling [31]. Another factor important in the field of physical therapy that justifies the performance of this test is the ability of the gait speed test to be coached and the health effect this creates, such as a 58% reduction in relative risk and a 17.7% reduction in the absolute risk of death [4]. In any case, some safety protocol and higher rest time between attempts must be taken into consideration, when the subjects have some hemodynamic risk condition, here the perception of effort is important [16],[21],[32].
Even though it is considered a valid tool in diagnostics, prognostics, relative decisions making for treatments, and following and monitoring interventions, the gait speed test is rarely used in practice. The analysis performed by Studenski [14],[33] mentions factors that could prevent its use: (1) a lack of normative references that confer an easy and direct interpretation to it; (2) the difficulty of adding new elements to the integral valuation, considering the little time and high workload of the health care centers where the healthcare professionals carry out their work; and (3) the physical space available. Regarding this last point, Guralnik noted that the trajectory length has to allow the test should be able to be used daily in the clinical setting [34].
In the study conducted by Peters et al. (2013), two different distance protocols of 4 and 10 m were compared to examine their reliability, and the researchers concluded that the reliability of both tests was excellent [18]. However, the 4-m test showed a lower level of concurrent validity compared to the 10-m test, causing the researchers to recommend a greater distance test [18],[19]. In this study, the same timed gait speed test distances from the Peters et al. Study (2013) were used. In this case, non-timed phases of equal distance were included in both tests, which may have affected the final results [18]. As far as we know, only the study by Phan-Ba et al. (2012) has compared the gait speed between the protocols with and without an acceleration phase (static start). The gait speed calculated is faster in those of dynamic start; in this case, the deceleration phases were not incorporated, contrasting with our study [35].
Authors such as Bohannon, Steffen (2002), Lusardi (2003), Fritz (2009)[36],[37],[9],[18],[19] also included phases of acceleration and deceleration as part of the protocol for measuring gait speed. However, the main reference to the use of these phases is the study conducted by Sustakoski et al. (2015)[8] who affirmed that the non-timed walking phase is one of the main factors that could alter the final results of the test. Standardized non-timed phases are not yet established, and some uncertainty exists over whether or not these phases are necessary. Nevertheless, allowing acceleration and deceleration to occur, may allow for a more accurate assessment of gait speed maximal and self-selected. Exclusion of a non-timed phase may introduce greater variability into gait speed measurement, which hinders the ability for the test to capture true change [20]. The authors also emphasize the importance of the type of walking surface used in the test and call upon clinic and researchers to make themselves aware of the different protocols used to measure gait speed properly.
Considerations for future research include the standardization of test instructions, such as start signals and verbal instructions given during the test, and perhaps the need to modify the test for participants with specific health conditions (maximum gait speed and comfortable gait speed). Here comes the functional reserve concept, where the type of step becomes very important. Bridenbaugh & Kressig (2011) perform five different walks for one 20-minute gait analysis session; three of those were single tasks: normal (self-chosen pace), slow (“slower than normal”) and fast (“as fast as possible”) walking. Normal walking provides us with baseline information about their mobility while slow walking can provide insights into the mediolateral stability. The difference between normal and fast walking provides information about their functional reserve. Also, measuring gait at all three speeds allows the normalization of certain biomechanical gait parameters [38].
Standardizing the protocols for measuring gait speed could provide more homogeneous results, allowing for greater comparisons and the extrapolation of results across different populations. Lyons et al. (2015) are one of the few authors who identified a limitation in these phases (acceleration and deceleration) and argued that it could explain the reason why the test of shorter length was less reliable [39]. On the other hand, there is a precedent in literature regarding the influence of these phases on the test, which is the study conducted by Wang et al. (2012)[40], where the researchers obtained similar results in groups of young adults and older persons (n = 71; Range 18 - 64 years old and n = 41; range 65 - 86 years old, respectively). In any case, the age spectrum is too broad, and the low sample of older adults is a limitation to compare results with other studies.
This study has some limitations that may restrict the generalizability of our findings. The primary limitation is that its observational design limits conclusions regarding cause and effect. While the variables associated with the previous instructions of the test are not defined, such as the specific type of footwear (subjects were only asked to wear comfortable footwear), we cannot determine whether changes in these variables will induce changes in the gait speed. Second, the sample was limited to community-dwelling older persons so the findings may not generalize to clinical populations in whom it may be equal or more relevant to measure gait speed as proxy measures as a vital sign for physical function. Third, one of the inclusion criteria was to use technical aids or not to use them, although, in reality, nobody indicated use it, if this had happened, there could be a possible external validity bias, which would make it difficult to extrapolate the results. Last, while the main focus of the study was the non-timed phases of the gait speed test (acceleration and deceleration), the omission of these for the comparison of reliability was not taken into account so that future researchers could take into consideration.
Conclusion
Our study results indicate that the ICC value was excellent in all cases. The number of attempts does not affect the results of gait speed. However and despite this, further analysis is required to conclude the same regarding the distance of the test (4- and 10-m). There is an insufficient agreement between the two tests to allow them to be used interchangeably in the independent dwelling-community older person. With that said, the aim of this study is not to promote one test above the other, but to highlight the fact that longer distances (10-m test) could be more appropriate in measuring the gait speed of this population. We underscore the idea that the tests could not be used interchangeably since the average difference is greater than the MDC95.
We suggest that further research is required to examine the environmental conditions especially regarding the effect on the acceleration and deceleration phases. It may be necessary to compare gait speed tests with a standard timed distance, but with variation in non-timed phases with the omission of one of the non-timed phases, starting or ending, and different distances when these are included.
Notes
Contributor Roles
LFH: Conceptualization, Formal analysis, Investigation, Methodology, Project administration, Supervision, Validation, Visualization, Writing – original draft, Writing – review & editing.
KCL: Conceptualization, Data curation, Formal analysis, Investigation, Methodology, Project, Validation, Visualization, Writing – original draft, Writing – review & editing.
Declaration of interests
The authors have completed the declaration of conflicts of interest of the ICMJE form and declare not having received financing for the completion of the report; the authors have not financial relationships with organizations that might have interests in the published article.
Financing statement
The authors declare that there were no external sources of funding.
From the editor
The manuscript was originally submitted in English. The journal has lightly edited the published version.
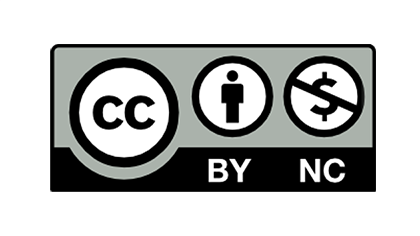