Clinical practice
← vista completaPublished on July 2, 2020 | http://doi.org/10.5867/medwave.2020.06.7950
Implications of COVID-19 (SARS-CoV-2) for the anesthesiological practice
Implicaciones del COVID-19 (SARS-CoV-2) para la práctica anestesiológica
Abstract
The purpose of this article is to review the characteristics of SARS-CoV-2, the clinical-epidemiological aspects of COVID-19, and the implications anesthesiologists when performing aerosol-generating procedures. A search of PubMed/MEDLINE, Scopus, SciELO, and Web of Science databases was performed until April 9, 2020, using the words: "COVID-19 or COVID19 or SARS-CoV-2 and anesthesiology or anesthesia". Forty-eight articles with information on the management of the patient in the perioperative period or the intensive care unit when suspected or confirmed SARS-CoV-2 infection were included. In general, the postponement of elective surgeries for no more than 6 to 8 weeks, depending on the clinical condition of the patients is recommended. In the case of urgent or emergency surgeries, we review the use of personal protection gear, as well as the recommended strategies for carrying out the procedure.
Main messages
- Given the recent pandemic of COVID-19, it is necessary to review the implications that the disease represents for anesthesiological practice, both for the management of patients and for establishing personal protection measures.
- This article provides a review of the information, recommendations, and guidelines for anesthesiology in the context of the pandemic.
- However, as it is a new disease, the information presented here can be reinforced or refuted as the available knowledge increases.
Introduction
COVID-19 is an infectious disease caused by the recently discovered coronavirus SARS-CoV-2, unknown until the viral pneumonia outbreak reported in Wuhan, China, in December 2019[1]. The disease outbreak grew substantially and spread throughout the world, infecting almost 2 million people and causing the death of more than 130,000 as of April 16, 2020[2].
SARS-CoV-2 enters mainly through the respiratory mucosa from droplets or aerosols of an infected person, or through contact with surfaces contaminated with the virus. In clinical practice, this transmission mechanism is a threat to health personnel, including anesthesiologists, who have a high probability of having direct or indirect airway contact when administering surgical anesthesia or performing aerosol-generating procedures (tracheal intubation and extubation, positive pressure ventilation, use of noninvasive mechanical ventilation and administration of high-flow oxygen, including coughing and vomiting as a consequence of these procedures) in patients potentially infected with SARS-CoV-2. The objective of this article is to review the characteristics of SARS-CoV-2, the clinical-epidemiological aspects of COVID-19, and the implications for anesthesiologists when performing aerosol-generating procedures.
Methods
We revied the origin, epidemiology, and characteristics of SARS-CoV-2 and the main clinical manifestations of the COVID-19 disease. Subsequently, a search of the scientific literature available in PubMed, Scopus, SciELO, and Web of Science databases, up to April 9, 2020, was carried out. The keywords "COVID-19 or COVID19 or SARS-CoV-2 and anesthesiology or anesthesia" were used and all articles published in English (regardless of design), whose summaries included information related to the anesthetic management of the patient in the perioperative period or the intensive care unit, with suspected or confirmed SARS-CoV-2 infection and COVID-19 disease, were included. Fifty-nine references and abstracts were located and evaluated by two independent researchers. Forty-eight articles that were eligible for inclusion were selected and evaluated in full text.
Review
Origin
The first reports of a new form of severe pneumonia in Wuhan, Hubei, China, occurred in December 2019[3]. The typical clinical symptoms of the first reported cases were fever, dry cough, dyspnea, headache, and pneumonia. In some cases, the disease proceeded to progressive respiratory failure secondary to alveolar damage and even death[4].
Samples from seven patients, who were admitted to the intensive care unit of Wuhan Jin Yin-Tan Hospital at the start of the outbreak, were analyzed at the Wuhan Institute of Virology for coronaviruses (the outbreak occurred in winter in a shellfish market, the same environment in which coronavirus infections related to severe acute respiratory syndrome, SARS-CoV). Five of the samples tested positive by polymerase chain reaction. A sample of bronchioalveolar lavage fluid was analyzed to identify the etiologic agent, using metagenomics with new generation sequencing and subsequent directed polymerase chain reaction. The viral agent had a 79.6% identity with the SARS-CoV sequence, so it was initially named as the 2019 novel coronavirus (2019-nCoV)[4]. The virus was recently renamed SARS-CoV-2 and the clinical picture as coronavirus disease 2019 (COVID-19)[1].
SARS-CoV-2
Coronaviruses are non-segmented positive-sense ribonucleic acid-enveloped viruses of the family Coronaviridae and of the order Nidovirales that are widely distributed in humans, other mammals (e.g., camels, cows, cats, and bats) and birds, causing respiratory, intestinal, hepatic and neurological diseases[3],[4]. Rarely, animal coronaviruses can infect humans and then be transmitted among people[5]. However, the high prevalence and wide distribution of coronaviruses, their great genetic diversity, their frequent genomic recombination and the increase in human-animal contact, increases the probability that new coronaviruses will appear periodically in humans, causing infections between species and occasional contagion events. Although most human coronavirus infections are mild, SARS-CoV-2, like SARS-CoV and Middle East respiratory syndrome coronavirus (MERS-CoV), are ß-coronaviruses, zoonotic in origin and may produce potentially fatal severe respiratory tract infections[4].
The complete sequence of a patient's SARS-CoV-2 was recorded and made available on GenBank (GenBank: MN908947) in January 2020. Following China, the Center for Disease Control and Prevention released the isolated virus sequences of various patients, with approximately 200 genomes so far available, representing a great genomic diversity of the virus in China and other populations[5]. Zhang et al.[6] analyzed the SARS-CoV-2 genotypes in different patients from various provinces in China, finding that it had mutated although the degree of diversification was small. Tang et al.[7] conducted a population genetics analysis of 103 SARS-CoV-2 genomes and classified them into two groups according to their envelope prevalence with type L in 70% of cases and type S in 30% of cases. Strains in type L appear to be derived from type S and are evolutionarily more aggressive and contagious[8].
So far, it is known that one of the mechanisms with potential clinical involvement is related to the renin-angiotensin-aldosterone system, a key system in human physiology. SARS-CoV-1 and SARS-CoV-2 interact with the renin-angiotensin-aldosterone system through the angiotensin-converting enzyme 2, an enzyme that physiologically counteracts or prevents activation of the renin-angiotensin-aldosterone system, but also functions as one of the viral receptors, so it has been proposed as a potential factor for virus infectivity. Both viruses activate the immune response and trigger secretion of inflammatory cytokines and chemokines in pulmonary vascular endothelial cells, so the cytokine "cascade" or "storm" has been postulated as the mechanism that causes organ failure[9].
On the other hand, Zhou et al.[10] carried out viral infectivity studies showing that SARS-CoV-2 uses angiotensin-converting enzyme 2 from humans, bats, civets, and pigs as an input receptor and that it does not use other coronavirus receptors such as aminopeptidase N and dipeptidyl peptidase 4. From the clinical point of view, these reports generated concern given the possible association of the use of renin-angiotensin-aldosterone system inhibitors (that could alter the angiotensin-converting enzyme 2 and modify its expression) with the severity in patients with systemic arterial hypertension and the use of such drugs. Some health systems went as far as to recommend the suspension of angiotensin-converting enzyme inhibitors and angiotensin receptor blockers on suspicion of SARS-CoV-2 infection. Moreover, initial clinical reports showed a high prevalence of systemic arterial hypertension among patients with SARS-CoV-2, including greater severity of the disease, with a higher proportion of patients requiring admission to intensive care units and mechanical ventilation, or who died. These observations led to hypothesize that medical management for this comorbidity, including the use of renin-angiotensin-aldosterone system inhibitors, could have contributed to the reported adverse results; although to date, the clinical molecular correlation is inconclusive[11],[12].
Epidemiology
The outbreak of the disease grew substantially and spread in a very short period through different countries as a consequence of human-to-human transmission. The World Health Organization (WHO) declared the COVID-19 epidemic a health emergency of international concern on January 30, 2020, with a total of 7,736 confirmed cases in China and 82 cases in 18 different countries[13]. Given that Wuhan is one of the cities with the most significant amount of travel in China (approximately 3,500 people travel by air on average daily), control measures were implemented and later extended to adjacent cities[14]. However, cases continued to increase rapidly worldwide, so the WHO declared COVID-19 a pandemic on March 11, 2020[15].
On February 5, 2020, in Yokoyama, Japan, the cruise ship Diamond Princess, with 3,711 passengers and crew, was quarantined for two weeks when a passenger with COVID-19 was reported after disembarking in Hong Kong. From March 16[16] to April 16, 2020[2], 712 people had been reported positive for SARS-CoV-2 infection, and 12 had died. Most of the infections occurred before or very close to the quarantine start date, which demonstrated the potential transmission of the virus, especially in confined sites. With the data reported up to February 21 (634 people infected), Mizumoto et al.[17] using statistical modeling estimated that the proportion of asymptomatic individuals among those who tested positive for SARS-CoV-2 was 17.9% (95% confidence interval: 15.5 to 20.2%).
As of April 16, 2020, the virus had infected 1 991 562 people and caused 130 885 deaths worldwide (Table 1)[2].
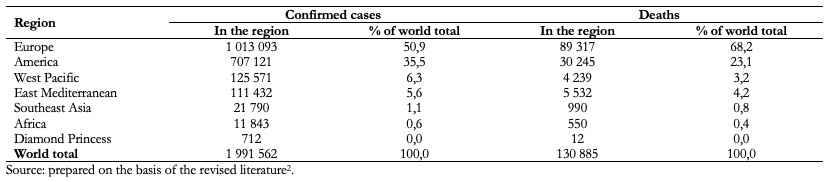
Clinical manifestations
Clinical symptoms include fever (83 to 98.6%), dry cough (47 to 82%), dyspnea (14 to 59%), myalgia or fatigue (11 to 44%), sputum production (20 to 28%), headache (6.5 to 16%), dizziness (9.4%), sore throat (5 to 17.4%), runny nose or nasal congestion (4%), hemoptysis (5%), loss of appetite (18 to 39.9%), nausea and vomiting (from one to 10.1%), abdominal pain (2.2%) and diarrhea (3 to 14%)[3],[9],[18],[19]. Olfactory dysfunction has recently been reported in 85.6% and gustatory dysfunction in 88% of patients in the European population[20].
Most cases have been classified as moderate (81%) since they did not present pneumonia or presented in a mild form, however, 14% of cases have been severe and 5% critical[21]. Patients requiring intensive care are older (over 65 years) and with a higher frequency of comorbidity such as systemic arterial hypertension (58.3%), diabetes (22.2%), cardiovascular disease (25%) and cerebrovascular disease (16.7%) (p <0.05)[19].
Laboratory findings of patients with COVID-19 are shown in Table 2. Comparing patients admitted to the intensive care unit to those not admitted to the intensive care unit, a higher leukocyte, and neutrophil count was observed, but a lower count of lymphocytes[19], higher elevation of prothrombin time and D-dimer[3],[19], higher creatine kinase myocardial band, lactic dehydrogenase, alanine aminotransferase, aspartate aminotransferase, total bilirubin, blood urea nitrogen and creatinine, as well as increased procalcitonin (p < 0.05)[19].
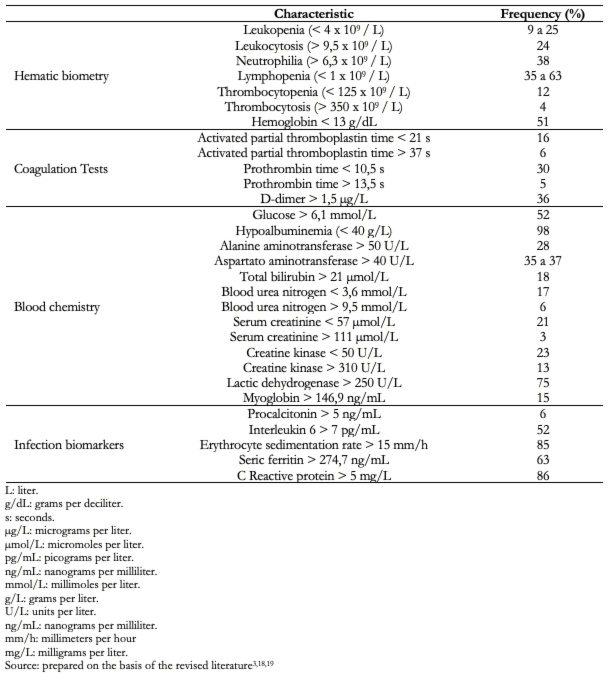
Qin et al.[22] also reported decreased B cells, T cells (particularly CD3+CD4+ T helper cells and CD3+CD8+suppressors) and natural killer cells, as well as increased neutrophil/lymphocytes and pro-inflammatory cytokine levels (interleukins -2R, IL-6, IL-8, IL-10, and tumor necrosis factor α), observing greater alteration in the most severe cases of the disease and associated with increased mortality in the case of the neutrophil-lymphocyte ratio[23].
Regarding radiological findings, computerized chest tomography images at hospital admission have shown bilateral lung involvement in 98% of cases[3]. Pure ground-glass opacity (77%) with reticular pattern and/or interlobular septal thickening (75%), subsegmental areas of consolidation (59%), and bronchogram (80%) have been reported. Other features include crosslinking, pleural effusion, small pericardial effusion, and lymphadenopathy[24].
The principal reported complications include progression to acute respiratory distress syndrome (19.6 to 29%), acute heart failure (7.2 to 12%), arrhythmia (16.7%), secondary infection (12%), acute renal failure (7%) and shock (8.7 to 7%)[3],[19]. Most patients have been treated with different antiviral drug schemes, antibiotics, glucocorticoids, alpha interferon, immunoglobulins, and antifungals[25]. In 26% of the cases, admission to the intensive care unit was necessary. The use of high-flow oxygen has been described in 11.1%, noninvasive mechanical ventilation in 44.4%, and invasive mechanical ventilation in 10 to 47.2%, requiring rescue therapy with extracorporeal oxygenation membrane in 5 to 23% of cases[3],[19].
The general prognosis has been towards recovery, although the total mortality rate has been reported at 2.3% with variations depending on age, from 8% in patients 70 to 79 years of age up to 14.8% in those over 80. In critically ill patients the reported mortality rate is much higher (49%), as well as in patients with cardiovascular disease (10.5%), diabetes mellitus (7.3%), chronic respiratory disease (6.3%), systemic arterial hypertension (6.0%) and cancer (5.6%)[21].
Transmission dynamics
Li et al.[26] reported a SARS-CoV-2 incubation time of 5.2 days (95% confidence interval: 4.1 to 7.0) and with data obtained up to January 4, 2020, estimated a growth rate on the epidemic curve of 0.1 per day (95% confidence interval: 0.050 to 0.16), with a doubling time of 7.4 days (95% confidence interval: 4.2 to 14), and an expected number of cases directly produced by a person in a population susceptible to infection (R0) of 2.2 (95% confidence interval: 1.4 to 3.9). Subsequently, another study showed similar data regarding incubation time[27], so a quarantine period of 14 days has been suggested. Regarding the R0 in more recent models, a value of 2.24 to 3.58 has been estimated[28], even as high as 6.47, if generations of viral transmission and intensive social contacts are taken into account[29].
The start and duration of the contagious period are not well defined. There are reports of asymptomatic infections, that is, the detection of viral ribonucleic acid without the presence of symptoms in 1% of cases[21], and pre-symptomatic infections with detection of the virus before the development of symptoms[30]. The SARS-CoV-2 ribonucleic acid has been detected in samples from the upper and lower respiratory system, blood, and feces, with a duration of several weeks, while in some patients, the virus has been isolated from samples of the upper respiratory system, bronchioalveolar lavage fluid, and stool cell cultures. It is still unknown whether other body fluids (vomit, urine, breast milk, or semen) from an infected person may contain viable and infectious SARS-CoV-2[5].
So far, it is known that SARS-CoV-2 is spread from person to person, mainly through droplets produced by the airways of infected persons that can reach directly, or through contaminated surfaces, the mouth, nose, and eyes, or by inhalation into the lungs. Transmission is more likely when the distance between people is less than two meters, making community transmission easy and feasible in various geographic areas, even without people knowing how and where they have become infected[5]. Van Doremalen et al.[31] showed that SARS-CoV-2 could remain viable and infectious for hours in aerosols and for days on surfaces (with greater stability on plastic and stainless steel than on copper and cardboard), depending on the inoculum.
In this way, transmission in health care environments is a threat both to patients who come to receive medical care and for health personnel. Wang et al.[19] reported the suspicion of hospital transmission as a mechanism of infection in 29% of the health professionals affected and in 12.3% of hospitalized patients. In contrast, Wu et al.[21] reported that of 44,672 confirmed cases in China, 3.8% corresponded to health workers, of which 14.8% presented critical illness, and of these, 1.96% died.
This situation increases concern about the presence of infections in health personnel despite the apparent use of personal protective equipment. However, it should be noted that health personnel may be exposed to contagion before the use of personal protective equipment. Therefore, the timely identification and isolation of suspected cases are very important[14]. In the case of anesthesiologists who are involved in procedures that generate aerosols (tracheal intubation, noninvasive ventilation, tracheostomy, cardiopulmonary resuscitation, mechanical ventilation before intubation, and bronchoscopy), it is important to review the different scenarios and protective measures that should be carried out to reduce the transmission of SARS-CoV-2.
Health personnel protection system
Personal protective equipment is an essential component of the healthcare personnel protection system, yet not the only measure, since the area for performing aerosol-generating medical procedures must also be adequately prepared, and strategies must be established to minimize their generation.
Table 3 shows the main measures or suggestions to take into account in the different areas of the healthcare personnel protection system, and Table 4 shows the levels of personal protective equipment for healthcare workers[5],[14],[32],[33],[34],[35],[36],[37],[38],[39],[40]. It is worth mentioning that these measures must be taken into account on a patient with suspected or confirmed SARS-CoV-2 infection, regardless of the type of anesthetic or surgical procedure to be performed. Furthermore, it is important to emphasize that no symptoms of COVID-19 in healthcare personnel should be minimized, so they should be immediately evaluated to rule out SARS-CoV-2 infection and establish timely and adequate management.
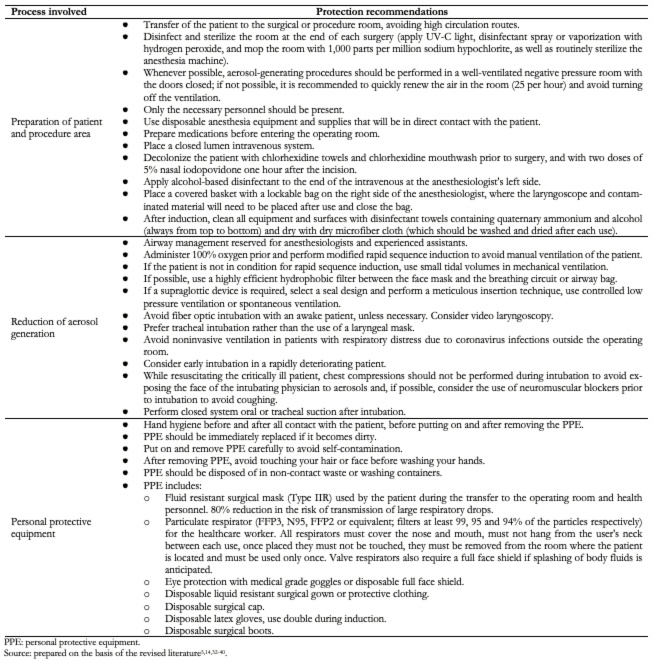
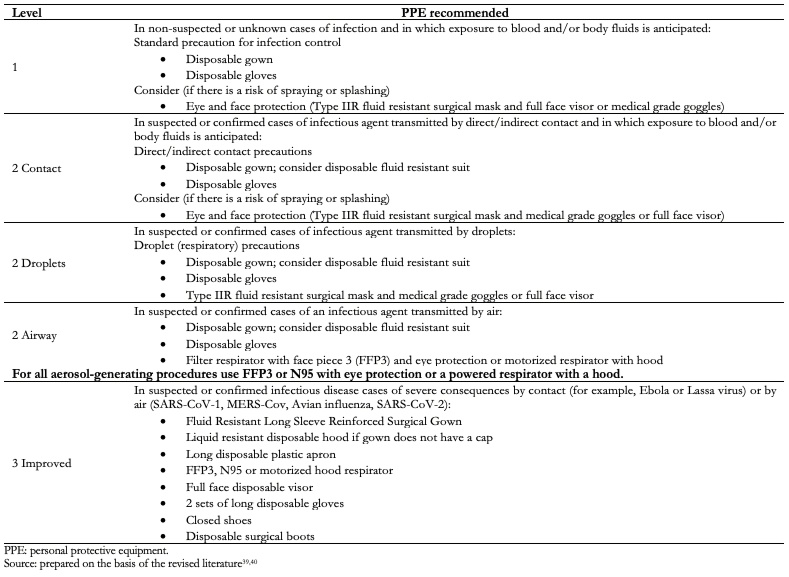
Elective and emergency surgery
Currently, the reorganization of surgical and anesthetic services is ongoing around the world. In addition to existing patients who require surgical management (sometimes urgent or short-term) with intensive postoperative care (major surgeries, including cardiac and oncological), there are also a large number of patients with suspected or confirmed COVID-19. They deserve hospital care and even admission to the intensive care unit[41]. Even though each society and institution has issued their recommendations, all have agreed on the postponement of no more than eight weeks (if possible and taking into account the characteristics of the patient and their disease) of all elective and essential surgeries, given etiopathological considerations related to surgical trauma, SARS-CoV-2 infection and the presence of comorbidity.
There is evidence of the redistribution of lymphocytes from the vascular area to the lymphatic tissue with subsequent lymphopenia, and the immune response produced by surgical trauma, by stimulating the secretion of various inflammatory mediators including cytokines, reactive oxygen species, nitric oxide, activating factor of platelets, growth factors, and eicosanoids, which in turn activate polymorphonuclear leukocytes, endothelial cells, macrophages, and lymphocytes, and may aggravate a SARS-CoV-2 infection or increase patients' susceptibility to infection[42].
Under normal conditions, some cytokines function as immunity regulators to limit damage or excessive inflammatory response, so there must be a dynamic balance between pro and anti-inflammatory cytokines to avoid organ dysfunction and infection and therefore reduce postoperative morbidity and mortality. Said balance could be altered in patients with immune compromisation associated with SARS-CoV-2 infection, characterized by an increase in the secretion of pro-inflammatory cytokines previously described. In particular, interleukin 6, whose levels have been observed to be significantly elevated (a marker associated with severity of critically ill patients, the occurrence of organ failure and myocardial dysfunction during sepsis) in patients with severe pneumonia[22].
Patients scheduled for surgery during the COVID-19 incubation period, although apparently healthy, are not free from SARS-CoV-2 infection and therefore have already started viral replication and associated immune changes. Lei et al.[43] reported that in 34 patients who underwent elective surgery during the SARS-CoV-2 incubation period, all developed short-term pneumonia after surgery, 44.1% required admission to the intensive care unit, and 20.5% died after admission to the intensive care unit, the main complications being acute respiratory distress syndrome, shock, arrhythmia and acute heart failure. Thus, surgery can accelerate or exacerbate the progression of COVID-19.
Although the SARS-CoV-2 patients admitted to the intensive care unit after surgery were older, underwent more complicated surgeries, and had a higher frequency of comorbidity[18], it is recommended that all patients scheduled for surgery have an isolation period of 14 days, or infection must be excluded in the patient, before being considered for elective surgery during the COVID-19 pandemic[43].
In the case of patients with cardiac surgery, alteration in the expression of the monocyte surface protein HLA-DR (involved in response to pathogens) and inhibition of monocytic and dendritic functions, factors associated with a longer stay in the ICU and higher mortality in cases of severe infection have been reported[42], which, added to the risk of SARS-CoV-2 infection, could be catastrophic for patients.
As for cancer patients, an increased risk of developing severe SARS-CoV-2 infection has been suggested, probably due to associated immunosuppression. Liang et al.[44] reported that 75% of the patients with neoplasm who underwent surgery or treatment one month before SARS-CoV-2 infection presented with severe disease.
On the other hand, some markers associated with a poor prognosis in cancer patients also are present in patients with COVID-19. This raises more concern, for example, in patients with colorectal cancer as a neutrophil/lymphocyte ratio > 2.8 increases the risk of postoperative complications at two years (hazard ratio: 5.36; 95% confidence interval: 1.95 to 14.90)[45], while in patients with COVID-19 an elevated neutrophil/lymphocyte ratio is an independent risk factor for hospital mortality[23].
Regarding urgent and emergent surgical procedures (including superficial and peripheral surgical procedures), as well as specific diagnostic procedures, these can be performed in available or reconditioned ambulatory surgery areas away from emergency services and intensive care. These include those related to trauma (fractures, dislocations, tendon repairs, compartment syndrome and drainage of abscesses or bruises), those related to cancer (diagnostic or therapeutic), urological procedures with the potential for progression to obstruction/infection or severe pain that warrant hospitalization, or even certain emerging intra-abdominal procedures (e.g., appendectomy and cholecystectomy). These procedures should be performed on patients without clinical suspicion of COVID-19 and, if possible, negative for SARS-CoV-2 infection confirmed by a polymerase chain reaction. If the patient is suspected or diagnosed with COVID-19, or if the procedure to be performed is life-endangering, it must be managed within the hospital operating room following specific protocols for each patient and condition[41].
Orotracheal intubation and ventilation
Approximately 3.2% of COVID-19 patients require invasive intubation and ventilation at some point in the course of the disease, particularly when hypoxemia increases despite receiving high-flow oxygen therapy, and positive pressure ventilation is insufficient. Even when elective surgeries are postponed, emergency surgeries (including cesarean sections) may arise in patients with suspected or confirmed COVID-19, which warrant general anesthesia with endotracheal intubation. Both situations require self-protection measures for healthcare personnel, as well as adequate preparation and management of patients' airway[46].
In general, the decision to intubate is immediate in the presence of cardiopulmonary failure or compromise/loss of the airway, however, in patients with acute respiratory failure and hypoxemia secondary to SARS-CoV-2 infection, close monitoring should be performed to identify signs of respiratory decline and initiate elective rather than emergent intubation[38]. Preparation for intubation should start when oxygen saturation is less than 93%, and the arterial pressure of oxygen/inspired oxygen fraction is less than 300 millimeters of mercury/%[46]. The decision to intubate is immediately made when there is no improvement in respiratory distress, tachypnea (frequency over 30 per minute), and reduced oxygenation (arterial pressure of oxygen/inspired oxygen fraction is less than 150 millimeters of mercury/%) after two hours with high-flow oxygen or noninvasive mechanical ventilation[47].
Meng et al.[46] suggest the acronym OH-MS MAID (Oxygen, Helpers [available helpers], Monitor, Suction, Machine, Airway supplies [video laryngoscope, direct laryngoscope, etc.], Intravenous access, Drugs), to facilitate the preparation of intubation in patients with suspected or confirmed COVID-19.
The administration of noninvasive ventilation three minutes before intubation has been suggested as a pre-oxygenation method before performing a modified rapid sequence induction[37],[48]. Cricoid compression or displacement is recommended when vocal cord exposure is difficult, or the patient's fasting time is unknown[37]. In extremely anxious patients, midazolam at doses of one to two milligrams may be considered, whereas intravenous lidocaine 1.5 milligrams per kilogram or more may be effective in suppressing cough during intubation[46],[49]. Etomidate (from 0.2 to 0.3 milligrams per kilogram) is recommended for induction in patients with hemodynamic instability and propofol (from one to 1.5 milligrams per kilogram) for stable patients.
Immediately after a loss of consciousness, the use rocuronium from 1 to 1.2 milligrams per kilogram, or succinylcholine from one milligram per kilogram is recommended[46],[50]. To suppress the laryngeal reflex and optimize intubation, fentanyl from 50 to 100 micrograms, sufentanil from 10 to 20 micrograms, or remifentanil from 2.5 micrograms per kilogram can be administered[46]. After intubation, it is suggested to verify its success by capnography, nebulization inside the tube, evaluation of thoracic movements, oxygen saturation and color of the patient's mucosa, and avoiding auscultation of the thorax[37],[46].
In case of failure of intubation in suspected or confirmed COVID-19 patients, no more than two attempts at intubation are recommended (modifying the position, equipment, and technique between attempts) given the risk of viral dispersion, so a laryngeal mask must be inserted and even tracheostomy considered with due precautions[37],[50]. If awake tracheal intubation is necessary, it should be performed by an expert, and intravenous sedation should be administered to minimize cough[50].
The use of small tidal volumes is recommended during induction until the patient is intubated. The guidelines for protective lung ventilation in patients with severe acute respiratory syndrome suggest a tidal volume less than or equal to six milliliters per kilogram of weight, a respiratory rate less than or equal to 35 per minute, a plateau pressure less than or equal to 30 centimeters of water, and positive pressure at the end of expiration greater or equal to five centimeters of water[46]. Prone ventilation is recommended for at least 12 hours a day to improve lung mechanics and gas exchange[46],[51].
Even though the use of high-frequency oscillatory ventilation has been suggested in patients with lung damage from viral infections, this strategy is not recommended in the case of SARS-CoV-2 infection, given the volume of aerosols it generates. Regarding extracorporeal membrane oxygenation, some cases of its use have been reported. However, its use is recommended only in centers with appropriate technical capabilities and experience[51].
Regarding extubation after general anesthesia or when the patient's clinical status warrants, it should be carried out similar to intubation, by expert anesthesiologists and with the minimum necessary personnel in the room, in addition to the use of personal protective equipment (including double glove placement)[14]. It has also been suggested that a wet gauze be placed over the patient's nose and mouth just before extubation and that intravenous lidocaine be used to prevent cough[47],[48]. After extubation, it is recommended to place a surgical mask on the patient and provide supplemental oxygen through a plastic mask.
General anesthesia vs. regional anesthesia
One of the most critical decisions the anesthesiologist must make is the type of anesthesia for each patient, given their clinical characteristics and possible postoperative results. In the face of the COVID-19 pandemic, this decision requires taking into account the safety of medical personnel involved in surgery and anesthesia.
The selection of general anesthesia involves the use of two aerosol-generating medical procedures, orotracheal intubation, and extubation, which increase the risk of cross-infection with an odds ratio of up to 6.6 for the transmission of acute respiratory infection from patients to health professionals[52].
On the other hand, both central and peripheral regional anesthesia, in addition to avoiding airway manipulation, produce better postoperative pain control, reduce opioid consumption, improve postoperative cognitive dysfunction and therefore reduce the incidence of nausea and vomiting (an aerosol generator that can potentially contaminate healthcare personnel)[33],[34]. In patients with COVID-19, whose lung function may be compromised by pneumonia and respiratory dysfunction syndrome, regional anesthesia reduces postoperative complications by not altering ventilatory mechanics. Current evidence in patients with SARS-CoV-2 infection shows that regional anesthesia has managed to maintain adequate ventilatory mechanics without increasing the need for postoperative respiratory care and that no deaths have been reported in patients in whom it was used (even in urgent procedures)[34]. Therefore, those patients whose surgery can be performed with regional anesthesia should be managed with this technique. Otherwise, general anesthesia should be administered, taking into account all risks and necessary precautions.
Regional anesthesia
For the performance of both central and peripheral regional anesthesia procedures, it should be noted that the most experienced anesthesiologist should perform the procedures, in order to avoid as much as possible the conversion of a regional technique to general anesthesia, as urgent intubation in the context of the COVID-19 pandemic may be more complicated than in the typical setting. Planning is an essential aspect, so the duration of anesthesia and surgery must be taken into account. It is better to perform planned intubation at the beginning of the procedure with all the protective measures and without haste than emergency intubation with more difficulties.
In patients with COVID-19, it is not recommended to reduce the dose of local anesthetics or to allow cerebrospinal fluid to flow, due to the risk of contamination of the personnel. It should also be borne in mind that the performance of additional procedures increases the risk of cross-contamination. In the presence of suspected or confirmed SARS-CoV-2 infection (in the community dispersal phase it should be assumed that all patients are positive) if postoperative intravenous analgesia can be used, peripheral blocks and the placement of epidural catheters should be avoided, since more contact with patients is required when monitoring this analgesic modality.
Spinal and epidural anesthesia
During the COVID-19 pandemic, several case series managed with subarachnoid block and/or epidural block have been reported, mainly emergency cesarean sections, although they are not the only reported procedures that have been performed under spinal anesthesia.
Chen et al.[53] reported 17 patients with COVID-19 who presented pneumonia data on chest tomography without thrombocytopenia but with significant hypotension in 86% of cases who underwent cesarean section with epidural blockade with the standard technique. No complications or secondary symptoms were observed in the postoperative period, and most were discharged between six and 13 postoperative days. Regarding healthcare personnel, they did not report any case of SARS-CoV-2 infection among the ten anesthesiologists who participated in the 17 cesarean sections.
Xia H et al.[54] reported a case of a subarachnoid block with the standard technique for an emergency cesarean section, where the patient with SARS-CoV-2 infection presented signs of pneumonia on chest tomography, oxygen saturation of 92-93% and normal coagulation tests. Surgery went smoothly, except for the presence of cough, transient hypotension, and tachycardia (greater than 138 beats per minute) during surgery. Postoperative analgesia was performed through the inserted epidural catheter. The patient and the newborn were negative for SARS-CoV-2 infection on the third and fifth day of surgery and none of the doctors who intervened in the procedure was infected.
Zhong Q et al.[55] reported the use of spinal anesthesia, specifically subarachnoid block, in 49 patients with clinical and radiological data of COVID-19 and confirmation of SARS-CoV-2 infection by reverse transcription-chain polymerase reaction, in 26.5% of cases in which emergency cesarean section or orthopedic surgery was performed. All of the patients presented mild respiratory symptoms, 53.1% fever, and none presented thrombocytopenia. Before spinal anesthesia, prophylactic antiemetics were administered; however, 6.1% of the cases presented vomiting. All of the patients had an adequate clinical and postoperative course since none of the patients developed severe pneumonia or died from COVID-19.
Regarding healthcare personnel, after administration of subarachnoid blocks in which 44 anesthesiologists participated, five of them were positive for SARS-CoV-2 infection. When analyzing the type of personal protective equipment they used, it was observed that four of the seven anesthesiologists who used category 1 personal protective equipment (57.1%) and only one of the 37 anesthesiologists who used category 3 personal protective equipment (2.7%) were infected, therefore, the use of category 3 personal protective equipment shows a relative risk reduction of 95.3% (95% confidence interval: 63.7 to 99.4).
In general, most of the authors agree that regional techniques are less risky for both patients and healthcare personnel, provided that the clinical conditions of each patient and personal protection measures are taken into consideration.
Peripheral nerve block
If ultrasound-guided peripheral nerve block is performed, all equipment must be protected (transducer, display, and keyboard). In the case of the transducer, plastic camera covers can be used, and transparent bags can be used to cover the fluoroscopes since, in addition to protection, it allows for proper manipulation of the controls and the image display.
Sedation should be administered judiciously (since patients with SARS-CoV-2 infection have limited lung function), and the use of full-face masks is recommended, taking care to use the lowest possible flow of oxygen to maintain adequate saturation. Since the dispersion distances of exhaled air increase with increasing oxygen flow (lateral distances of 0.2; 0.22; 0.3 and 0.4 m from the sagittal plane during oxygen supply at four; six; eight and ten liters per minute respectively), and always monitoring expired carbon dioxide.
The use of nasal cannulas is not recommended due to possible aerosolization with oxygen flow[56]. As much as possible, peripheral blocks that can reduce lung capacity should be avoided, such as interscalene blocks that can potentially cause diaphragmatic hemiparesis or those that can cause pneumothorax, including supraclavicular block and infraclavicular block. If it is decided to use them, the risk-benefit ratio must be assessed, reducing the dose, volume or both, of local anesthetics—and always ultrasound-guided to not affect the ventilatory mechanics. Likewise, it should be taken into account that the usual complications of these procedures may arise and that airway manipulation may be required, so the necessary material and equipment must be available.
Conclusions
The COVID-19 pandemic has led to a rethinking of health systems, as currently, the number of cases is steadily increasing without sufficient hospital capacity, supplies, or human resources for their care.
Anesthesiologists, being experts in airway management, on the one hand, face an overload of work with the constant increase in patients infected with SARS-CoV-2 that warrant intubation and mechanical ventilation, and, on the other, patients with pathologies that due to their severity warrant short-term, emergency or urgent surgical interventions. However, even when clinical activities become strenuous, health personnel have not stopped transmitting their experience and the results of the constant clinical and molecular research that they carry out every day to attempt to improve patient care, for patients suffering from a disease, which was unknown until a few months ago, and which in certain cases can be fatal.
The knowledge available so far has led to the recommendation to postpone elective surgeries for no more than six to eight weeks according to the clinical conditions of the patients, and in the case of urgent or emergency surgeries, to establish a series of self-protection strategies for healthcare personnel, and for optimal patients care and management, given the risk involved in performing some medical procedures.
No doubt that new evidence emerging every day throughout the world will be most useful for healthcare professionals who face, or are about to face, similar situations, and each new experience and each discovery can either reinforce or refute the currently available guidelines and recommendations.
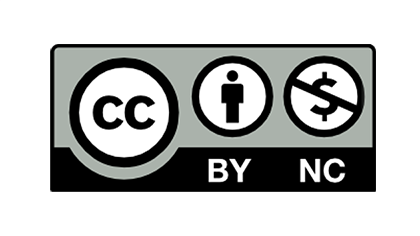