Critical appraisals
← vista completaPublished on August 14, 2015 | http://doi.org/10.5867/medwave.2015.07.6212
Effects of high intensity interval training versus moderate intensity continuous training on the reduction of oxidative stress in type 2 diabetic adult patients: CAT
Efectividad del entrenamiento interválico de alta intensidad comparado con entrenamiento continuo de moderada intensidad en la reducción de estrés oxidativo de pacientes adultos con diabetes mellitus tipo 2: CAT
Abstract
INTRODUCTION Oxidative stress is caused by an imbalance between an excessive production of reactive oxygen species and/or a deficiency in the level of endogenous and exogenous antioxidant defenses. The presence of reactive oxygen species in large concentrations and for long periods is associated with the occurrence of various diseases, including type 2 diabetes mellitus. Exercise represents an effective means for the prevention and treatment of type 2 diabetes mellitus, and is also able to reduce long-term oxidative stress levels. High-intensity interval training has shown to be an efficient and viable option for type 2 diabetes mellitus control. In turn, high-intensity interval training seems to have positive effects on oxidative stress levels by increasing levels of endogenous antioxidants.
OBJECTIVE To assess the validity and applicability of the results regarding the effectiveness of high-intensity interval training compared to moderate intensity continuous training to reduce oxidative stress in patients with type 2 diabetes mellitus and to answer the following question: In adults with type 2 diabetes mellitus, can the method of high-intensity interval training, compared to moderate intensity continuous training reduce oxidative stress levels?
METHODS We performed a critical analysis of the article "Continuous training vs Interval training in glycemic control and macro and microvascular reactivity in patients with type 2 diabetes".
RESULTS No statistically significant differences were observed in concentrations of superoxide dismutase in any of the experimental groups. Only in the interval group a decrease in malondialdehyde regarding control group and baseline (p<0.05) was observed. In addition, only in the interval group there was an increase in glutathione peroxidase compared to the group of continuous aerobic training and baseline (p<0.05). Nitric oxide showed a significant increase regarding the control, continuous aerobic group and baseline (p<0.05) in the interval training group.
CONCLUSIONS Despite the fact that both training groups show improvements over markers of lipid profile and fitness, high intensity interval training has shown to be more effective in the normalization of oxidative stress, impacting positively on the concentration of pro-oxidant markers and antioxidants.
Introduction
1. Oxidative stress
Throughout evolution, living organisms have adapted by using different elements found in the environment. One of these elements, vital for survival, is oxygen (O2) [1]. Oxygen is used by different types of cells in our body during production of energy in the electron-transport chain located in mitochondria. Different macronutrients, such as carbohydrates, lipids and proteins, are metabolized via different enzymatic reactions known as "intermediary metabolism" in order to produce energy in the form of adenosine triphosphate (ATP) with water (H2O) and carbon dioxide carbon dioxide (CO2) [1]. In this process, oxygen participates as a terminal electron (e-) and proton (H+) acceptor.
Oxygen is highly reactive and can be lethal in high concentrations. During energy production in electron-transport chain, some electrons may be released from certain complexes and accepted nonspecifically by oxygen, leading to the formation of molecules known as reactive oxygen species or reactive oxygen species (ROS) [2]. Some of these molecules are superoxide anion (O2•-), hydrogen peroxide (H2O2), and hydroxyl radical (•OH), which are formed by the following reactions [2]:
Reaction 1: O2+ e-→ O2•-
Reaction 2: O2•- + e-→ H2O2
Reaction 3: H2O2 + e-→ •OH
Reaction 4: •OH + e-→ H2O
Superoxide anion and hydroxyl radical are free radicals since they have an unpaired electron in their orbitals. While hydrogen peroxide is a reactive component, is not strictly considered a free radical [2].
High concentrations of reactive oxygen species can be dangerous as they can indiscriminately react with any molecule. These harmful effects can affect various cell components such as membrane phospholipids, proteins, various enzymes, and even to DNA [3]. There are various enzymes that produce reactive oxygen species such as xanthine oxidase (XO), NADPH oxidase, endothelial nitric oxide synthase (eNOS), cytochrome P450, etc. [4],[5],[6]. The xanthine oxidase can catalyze the oxidation of hypoxanthine to xanthine, besides fulfilling an important role in the catabolism of purines in some species, including humans. In turn, xanthine oxidase catalyzes the oxidation of xanthine to uric acid. In both processes superoxide anion is generated as shown in the following reactions:
Hypoxanthine + O2 → Xanthine + O2•-
Xanthine + H2O + O2 → Uric acid + O2•-
Moreover, NADPH oxidase catalyzes the reduction of a particle of oxygen to generate superoxide anion, using NADPH as an electron source. This enzyme has a bactericidal function in activated neutrophils, in which large amounts of toxic superoxide anion and other important reactive oxygen species are generated. The nitric oxide synthase (NOS) are a family of enzymes that convert L-arginine amino acid into L-citrulline and nitric (NO) oxide. His best known function is the regulation of vascular tone through nitric oxide. There are three isoforms of nitric oxide synthase: neuronal nitric oxide synthase (nNOS), endothelial nitric oxide synthase (eNOS) and inducible nitric oxide synthase (iNOS). Endothelial nitric oxide synthase can be uncoupled in the absence of L-arginine. In this state, the endothelial nitric oxide synthase can produce superoxide anion instead of nitric oxide [2].
In mammals has been observed that in rest conditions, between 0.15 and 5% of total oxygen consumed by the cells become reactive oxygen species [7]. Given this situation, living things have developed different ways to decrease reactive oxygen species, in order to avoid cellular damage. This endogenous antioxidant response allows to maintain optimal reactive oxygen species levels for life and is based on the action of various antioxidants, such as superoxide dismutase (SOD), catalase, glutathione peroxidase and glutathione reductase [6] enzymes.
There are different varieties of superoxide dismutase (SOD): SOD-Cu, Zn-SOD and Mn-SOD. The Cu-SOD and Zn-SOD enzymes are located in the cytosol, nucleus and mitochondrial intermembrane space [8]. Meanwhile, the Mn-SOD is found in the mitochondrial matrix. As the name implies, superoxide dismutase catalyzes the dismutation of superoxide anion, generating hydrogen peroxide and oxygen through the following reaction [2]:
O2•- + O2•- + 2H+→ H2O2 + O2
Even though the superoxide dismutase produces hydrogen peroxide, this reactive species that is less toxic superoxide anion. The decrease superoxide anion reduces the likelihood of forming hydroxyl radical. Further, hydrogen peroxide can be metabolized by catalase. This protein is another antioxidant enzyme present in all tissues, especially in hepatocytes and erythrocytes. At the cellular level it is located in mitochondria and peroxisomes, except erythrocytes where it is located in the cytosol. Catalase is capable of converting hydrogen peroxide into oxygen and water through the following reaction [2]:
H2O2+ H2O2→ O2+ 2H2O
Glutathione peroxidase catalyses the reduction of hydrogen peroxide at the expense of glutathione, converting the reduced glutathione (GSH) into oxidized glutathione (GSSG) and forming water by the following reaction [2]:
2GSH + H2O2→ GSSG + 2H2O
Oxidized glutathione is reduced by the action of glutathione reductase, which uses NADPH as a reducing equivalents donor, through the following reaction [2]:
GSSG + NADPH + H+→ 2GSH + NADP+
In addition to these enzymes, there are various exogenous antioxidant molecules, usually from the diet, such as vitamins E, A, and C contributing to decreased reactive oxygen species [2],[6].
When there is an excessive production of reactive oxygen species and / or a deficiency in the level of endogenous and exogenous antioxidant defenses, a state of imbalance called oxidative stress [2] occurs. One way to assess the damage by oxidative stress is by measuring malondialdehyde (MDA). This compound is synthesized as the product of peroxidation of polyunsaturated fatty acids present in the cell membrane and other phospholipids by the action of free radicals and reactive oxygen species. For this reason, the plasma concentration of malondialdehyde is proportional to oxidized polyunsaturated fatty acids, being a good indicator of lipid peroxidation [9].
Despite having toxic effects on the body in high concentrations, it should be noted that reactive oxygen species in normal concentrations can act as beneficial modulators in several physiological processes. These functions include cell growth [7],[10],[11], increased transcription of mitochondrial DNA [8], heart contractility [12], improvement in glucose uptake in working muscle [13], mild cardio protective effects after periods of ischemia or exercise [14],[15], increasing the overall antioxidant capacity of the organism [5],[10],[16], among others.
2. General epidemiological aspects
The presence of reactive oxygen species at high concentrations and for prolonged periods of time is associated with the occurrence of various diseases such as hypertension [4], insulin resistance and diabetes [17]; sarcopenia [18], overweight and obesity [19],[20]; metabolic syndrome [21], cancer and cachexia [22]; among others. In Chile there is a high prevalence of these diseases, primarily associated with a sedentary lifestyle status and poor nutrition (Table 1).
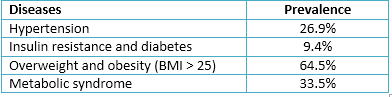
Therefore, it is essential to strike a balance between pro-oxidants and antioxidants factors, such that the reactive oxygen species behave as modulators and no pathological factors [11].
2.1 Type 2 diabetes mellitus and oxidative stress
Type 2 diabetes mellitus is one of the most prevalent chronic non-communicable diseases in Chile [23] and worldwide [24]. There is in turn a studied association between oxidative stress, insulin resistance and type 2 diabetes mellitus [17],[25],[26]. This is because in chronic conditions of hyperglycemia and / or hyperlipidemia high concentrations of reactive oxygen species are produced. This situation leads to a progressive deterioration in the normal function of β cells in the pancreas, thus affecting the synthesis and secretion of insulin [26].
Moreover, in cases where the type 2 diabetes is related to obesity, the signaling pathways of insulin may be affected by changes in conformation of insulin receptor substrates. Thus, functionally it alters the insulin receptor and translocation of glucose-dependent insulin transporter, GLUT-4, is decreased [27].
3. Effects of physical exercise on the production of reactive oxygen species.
During physical exercise there is a redistribution of blood flow due to an increase in metabolism of the active cells, particularly in skeletal muscle fibers. In addition, there is less blood flow in the visceral organs. While there is an increased blood flow to muscles, they may also experience brief periods of hypoxia, followed by periods of reperfusion. This is due to that muscle contractions greater than 50% of muscle functional capacity are capable of generating a short vascular occlusion [28]. This situation can lead to a paradoxical increase in the production of reactive oxygen species mitochondrial because, in the absence of oxygen, complex III of the electron-transport chain is able to generate superoxide anion in a controlled way [6],[8]. The superoxide anion would give rise, in turn, to other reactive oxygen species, being hydrogen peroxide the main intracellular signaling mediator (second messenger) [29].
On the other hand, during recovery period there is a post-exercise inflammatory process, which also leads to the generation of reactive oxygen species. This process would be mediated in part by activation of leukocytes, which induce the reaction between oxygen and NADPH oxidase to form superoxide anion [5]. It was observed that during muscle contraction adjacent endothelial xanthine oxidase plays an important role in the production and release of superoxide anion, compound that would be involved in muscle contraction force [30],[31]. There is a muscular hypoxia during high intensity exercise since the metabolic demands of the active muscles exceeds the oxygen flow [8]. This situation returns to normal in breaks, existing a reperfusion period of reperfusion in active tissues [32].
Reactive oxygen species play an important role during the exercise because it has been observed that muscle fiber increases its sensitivity to intracellular calcium (Ca2+), being able to produce stronger contractions both cardiomyocytes and skeletal muscle fibers [33]. Immediately after a session of high-intensity interval training , there is an increase in reactive oxygen species compared to 24 hours after exercise, independently of the physical condition of the subject [16]. Reactive oxygen species produced during and after a workout session, activate different intracellular signaling pathways associated with gene regulation processes. These processes would be mediated by other transcription factors such as nuclear factor KB (NF-KB), activator protein-1 (AP-1), signal transducer and activator of transcription 3 (STAT3), and hypoxia-inducible factors (HIF) [5],[6],[8],[34],[35]. NF-KB is a redox sensor which regulates gene expression of inflammation and cell growth factors [8],[10],[36] and increases in Mn SOD [35]. Likewise, AP-1 is another intracellular redox state sensor which regulates the expression of several genes related with responses to stress, growth, cell differentiation and increases Mn SOD [8],[35]. STAT3 regulates anti apoptotic, cell proliferation and differentiation signals; skeletal muscle regeneration and cardioprotection. STAT3 activation is induced by inflammatory markers such as cytokines, interleukin 6 (IL-6) and reactive oxygen species produced during exercise [37]. In cardiomyocytes, the STAT3 regulates ischemia-induced protection [37]. Hypoxia-inducible factor (HIF-1α and HIF 1-β) mediates transcriptional activation of several genes such as erythropoietin, vascular endothelial growth factor, among others [8].
3.1 High-intensity interval training
High-intensity interval training (HIIT) is a fractionated method derived from the “interval training”, which is widely used in athletics training [32],[38]. For this reason, the high-intensity interval training usually use aerobic-cyclical exercises such as running, cycling and others [32],[39]. However, there are some new proposals based on neuromuscular system development and which use mainly strength exercises [38],[39].
This training method is characterized by alternating short bouts of high intensity exercise with successive periods of recovery. Generally, efforts are performed with an intensity above the anaerobic threshold, which fluctuates in an estimated range between 80 and 95% of maximum heart rate, lasting 10 to 15 seconds until 4 or 5 minutes [40]. Likewise, recovery periods may reach an approximate intensity of 40-50% of maximum heart rate and a duration similar to or slightly above stress periods [38]. The amount, duration and intensity of intervals, in addition to the chosen method of exercise, will depend on the fitness and objectives of the individuals [32],[39].
It has been observed in experimental studies with human models, that after an intervention of three weeks of high-intensity interval training in “sprint-mode” (i.e. 4-6 intervals of 30 seconds at maximum intensity, 4 minutes of rest between each interval), with a frequency of three sessions per week, there is a significant improvement in the total antioxidant capacity in trained subjects [16].
3.2 Moderate-intensity continuous training
Moderate-intensity continuous training (MICT), also known as continuous or aerobic training, is a method widely used for endurance development, which is characterized by performing efforts of medium intensity (between 40 and 70% of maximum heart rate) and might be extended from 15 minutes to several hours [38]. Usually, it is recommended that this type of exercise should last at least 30 minutes. Typically, the means of exercising employed for this type training are aerobic-cyclical such as swimming, cycling or running. Currently, the exercise prescription for patients who have insulin resistance or type 2 diabetes, focuses on this type of training [41].
The popularization of high-intensity interval training as preparation method for therapeutic purposes is an issue that, from the point of view of medicine based evidence, still requires further support. For this reason, and considering the relationship between oxidative stress and type 2 diabetes mellitus prevalence is that the results of randomized clinical trial "Continuous vs interval training on glycemic control and macro- and microvascular reactivity in type 2 diabetic patients" will be analyzed [42]. This study aims to determine the effectiveness of high-intensity interval training compared to moderate-intensity continuous training in reducing oxidative stress in adult patients with type 2 diabetes mellitus.
Study analyzed
Mitranun W, Deerochanawong C, Tanaka H, Suksom D. Continuous vs interval training on glycemic control and macro- and microvascular reactivity in type 2 diabetic patients. Scand J Med Sci Sports. 2014 Apr;24(2):e69-76. doi: 10.1111/sms.12112. Epub 2013 Sep 17.
Type of study: randomized controlled clinical trial
Study characteristics
Randomized controlled trial which investigates the effects of aerobic interval training compared to continuous aerobic training on fitness, glycemic control and micro and macrovascular reactivity in elderly patients diagnosed with diabetes mellitus type 2. Forty three subjects were randomly allocated in three groups: aerobic interval training, continuous aerobic training, and sedentary. The exercise training intervention for the experimental groups (aerobic interval training and continuous aerobic training) consisted of training on a treadmill for 12 weeks with three sessions per week. Planning considered three training phases, which had the same volume and intensity for both groups. Health-related physical fitness, vascular reactivity and blood biochemistry data of patients was measured before and after intervention.
Objective
To investigate the effects of interval aerobic exercise training on glycemic control, health-related physical fitness, and micro- and macrovascular reactivity in older patients with type 2 diabetes.
Inclusion criteria
- Patients diagnosed with type 2 diabetes mellitus.
- Adults between 50-70 years.
- Baseline glycosylated hemoglobin (HbA1c) values of 53 and 75 mmol/mol (7 to 9%).
- No previous exercise training in the last 6 months.
- Postmenopausal women.
Exclusion criteria
- Patients diagnosed with nephropathy, retinopathy and/or severe neuropathy associated to diabetes.
- Patients diagnosed with severe cardiovascular or cerebrovascular diseases.
Intervention and comparison
The eligible participants were randomly allocated into three groups:
- Interval aerobic training (INT).
- Continuous aerobic training (CON).
- Sedentary control (SED).
Training plans for experimental groups considered three weekly sessions during a period of 12 weeks. In addition, planning contemplated a progressive adjustment of loads, represented in three training periods or phases, which are detailed in Table 2.
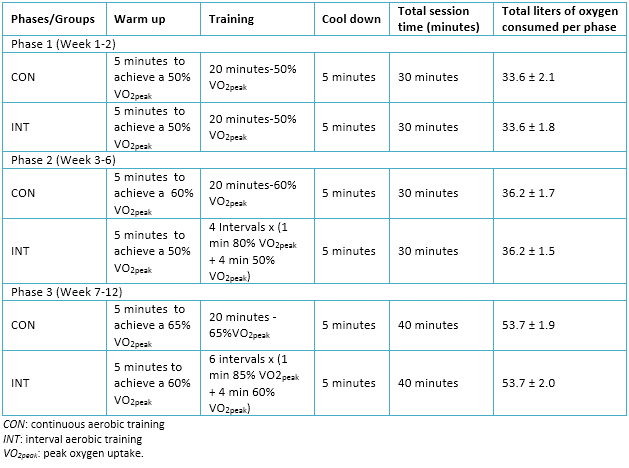
Study outcomes
Health-related physical fitness
Body composition was evaluated using a bioelectrical impedance analyzer (In Body 220, Biospace, Seoul, Korea). The maximum oxygen consumption was assessed directly with the system cardiopulmonary gas exchange (Cortex) through the modified Bruce protocol on treadmill. Heart rate was measured with a heart rate monitor (Polar Team 2 Pro, Polar Electro Inc., Lake Success, NY, USA). Muscle strength was assessed using the method of maximum repetition in the following exercises: leg extension, knee flexion, chest pressure and pulldowns. The measurement was carried out with such force Nautilus machines. Sit-and-reach test was used to assess the flexibility of the trunk
Blood biochemistry data
- Markers of oxidative stress: erythrocyte malondialdehyde concentration was measured with the method of thiobarbituric acid reactive substances.
- Bioavailability of nitric oxide: nitric oxide, according to the estimates of total concentrations of nitrite and nitrate, was measured in plasma with assay kit (colorimetric assay kit nitric oxide, PromoKine, Heidelberg, Germany).
- Markers of antioxidant defense: the level of glutathione peroxidase in erythrocytes was measured with assay kit (Ransel, Randox Laboratories Ltd., Antrim, United Kingdom). The concentration of superoxide dismutase was measured in erythrocytes assay kit (Ransod, Randox Laboratories Ltd).
- Glycemic and lipid profile: the concentration was measured in fasting blood glucose, glycosylated hemoglobin (HbA1c), lipid and lipoprotein (HDL and LDL), insulin and von Willebrand factor (vWF). It was calculated using equation [fasting glucose (mg / dL) × insulin level (uU / ml) / 405].
Vascular reactivity
The characteristics of the brachial artery was evaluated with ultrasound equipment (CX50, Philips, Andover, MA, USA), using the technique of arterial occlusion in the right forearm. The mean blood velocity Doppler pulsed mode was measured. Data evaluated were brachial base diameter, maximum brachial diameter, flow-mediated dilation, maximum diameter, shear rate at rest, vascular resistance (was calculated as the mean arterial pressure / brachial blood flow), vascular conductance (calculated as brachial blood flow / mean arterial pressure), skin blood flow at rest, maximal skin blood flow, and skin continuous flow ratio of maximal (were measured in the right wrist with a laser Doppler flowmetry).
Results
45 individuals who participated in the study, which were randomly assigned in their respective groups, only two did not complete 80% of the training program so they were excluded from statistical analysis. The levels of body fat and resting heart rate decreased, while the lower limb muscle strength increased (all p <0.05) in both continuous aerobic training group and the interval aerobic training group. Fasting blood glucose decreased ( p <0.05) in both exercise groups, but glycosylated hemoglobin levels decreased (p <0.05) only in aerobic interval training group. Maximum aerobic capacity, flow-mediated dilation and skin reactive hyperemia significantly increased in both exercise groups. However, the magnitude of improvement was greater in the group of aerobic training interval.
Only in the interval aerobic training group significantly decreased malondialdehyde concentration in erythrocytes and Von Willebrand factor also observed an increase in plasma glutathione peroxidase and nitric oxide (all p <0.05).
Appraisal
About validity
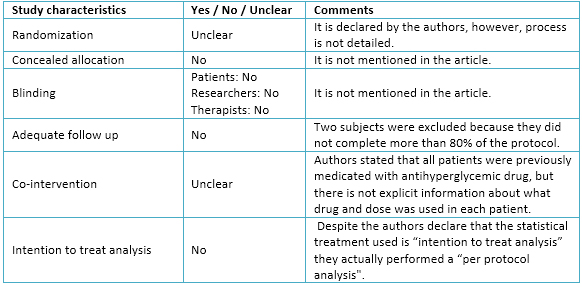
The authors declare that there was a randomization in the present trial. Moreover, it is possible to observe that there was a fairly homogeneous distribution among the three experimental groups. However, omission of details about process and methods used in randomization might imply bias in the sample selection.
Regarding allocation concealment and blinding, they are not mentioned in the article. This situation may lead to raise the selection bias of the sample, and eventually, produce performance and detection bias.
With regard to follow-up, the authors decided to exclude from the final analysis all patients who did not complete at least 80% of all training sessions. According to this criterion, two subjects were excluded (one from interval aerobic training and other continuous aerobic training). Despite this, the rate of adherence to exercise program was relatively high, whereby, attrition bias would not necessarily occur.
Authors stated that all patients were medicated with antihyperglycemic drugs, before physical exercise intervention, and that their doses were unchanged during the study. However, there is not specific data about which antihyperglycemic drugs were used by each patient nor if there were differences between doses during the intervention.
Statistical analysis used by authors was "per protocol" because they only considered data from those patients who completed more than 80% of the intervention. By performing this type of statistical analysis they do not consider patients who, for various reasons, might drop out of a training plan in reality. Likewise, only considering those patients who successfully complete the proposed program by researchers, the effects of different types of training could be overestimated. Because the decision of not considering this data may affect the trial outcomes, attrition bias could occur. The details are seen in Table 3.
About the results
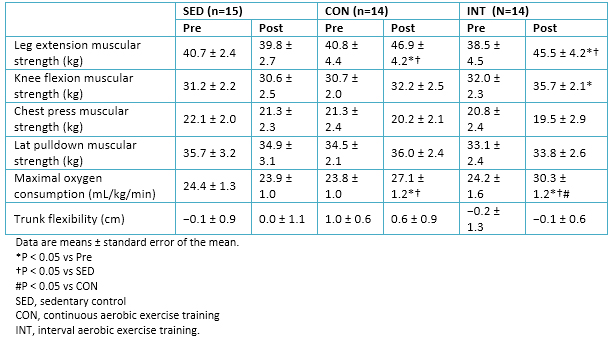
A significant decrease in systolic blood pressure was observed in the interval aerobic training (INT) group but not in the continuous aerobic training (CON) group . Leg extension strength increased in both groups, although the knee flexion strength only increased in the INT group. Measurements of strength in the upper body muscles were included to gain insight into the cross-transfer effects. Upper body strength, as assessed by bench press and lat pull downs, did not change in all the groups.
Maximal oxygen consumption increased in both exercise groups; the magnitude of increase was greater in the INT group than in the CON group. No changes in physical characteristics and physical fitness measures were observed in the sedentary control group (Table 4)
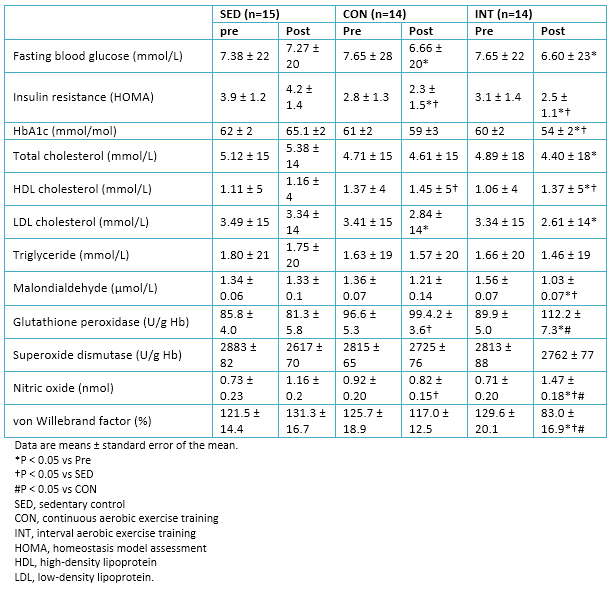
A significant decrease in fasting blood glucose and insulin resistance (HOMA) in both exercise groups was observed. Only in the interval aerobic training group there was a significant decrease in glycated hemoglobin levels. We can compare these results with those of other similar studies in diabetes mellitus type 2 patients, which affirm the significant and immediate reduction in blood glucose after a session of high-intensity interval training, as in effect long period following a program training [43].
Lipid profile improved significantly in both groups (continuous aerobic training and interval aerobic training), revealing the positive effects of moderate-intensity continuous training and high-intensity interval training in the regulation of lipoprotein metabolism.
In relation with factors associated with oxidative stress, a significant decrease in the levels of malondialdehyde was observed in the interval aerobic training group post intervention and in comparison with sedentary group. Furthermore, increased levels of von Willebrand factor, nitric oxide and glutathione peroxidase was presented (Table 5). Other studies had already shown an increase in glutathione peroxidase after a single session of high-intensity interval training in healthy subjects, with a maintaining of these levels until several hours later the effort [16],[44]. This evidence could complement and justify the improvement in the levels of glutathione peroxidase, after undergoing a period of training of high-intensity interval training.
It would be interesting to analyze the benefits of high-intensity interval training in other markers such as antioxidants catalase, which in healthy subjects has shown good results after high-intensity interval training sessions [16],[44], or also its effects on oxidative stress markers such as palmitoyl-2- (5-oxovaleroyl) -sn-glycero-3-phosphorylcholine and 1-palmitoyl-2-glutaroyl-sn-glycero-3-phosphorylcholine, which have declined after undergoing exercise programs [45].
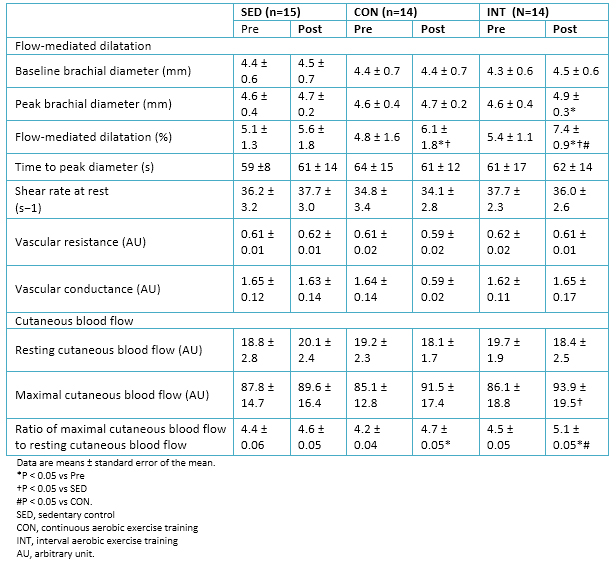
No significant changes in cutaneous blood flow were observed, both maximal and at rest, in either group. However, the ratio of maximal cutaneous blood flow to resting cutaneous blood flow (i.e., cutaneous hyperemia) increased in both exercise groups, but was significantly higher in the interval aerobic training group.
Similarly, flow-mediated dilation improved significantly (P <0.05) in both exercise groups after the intervention. However, the increase in flow-mediated dilation was significantly higher in the interval aerobic training group compared to the group of continuous aerobic training. Details in Table 6.
At baseline, the glycosylated hemoglobin was significantly related to maximal oxygen consumption and flow-mediated dilation. Nitric oxide concentrations were related to the flow-mediated dilatation (r = 0.69). Analyzing the pooled population, it was observed that changes in glycosylated hemoglobin levels showed a correlation with changes corresponding to the maximum oxygen consumption (r = -0.74) and flow-mediated dilatation (r = -0.72).
About applicability
Metabolic diseases are caused by various factors. However, most of them converge in inflammatory processes and in generation of chronic oxidative stress [4],[17],[18],[19],[20],[21],[22].
Currently in Chile, treatment of type 2 diabetes mellitus primarily includes drug prescriptions and nutrition interventions for glycemic control, assigning a complementary role to exercise. However, there is strong evidence about the importance of physical exercise in prevention and treatment of diabetes mellitus type 2 [46].
According to guidelines of Ministry of Health from Chile, adequate exercise modality for type 2 diabetes mellitus patients should put particular emphasis on moderate-intensity continuous training for endurance development, and “1x2x3 method” for strength development [41]. There is evidence regarding effectiveness of moderate-intensity continuous training in glycemic control, reduction of visceral adipose tissue and reducing blood triglycerides in patients with type 2 diabetes mellitus [46]. Nevertheless, in recent years it has been observed that high-intensity interval training has similar effects to moderate-intensity continuous training in relation to endurance development [47],[48], which can also become an intervention to control type 2 diabetes mellitus [49],[50]. Applicability of both methods depends largely on good equipment, professional skills of who are in charge and clinical profile of patients. Regarding equipment, we recommend using cycle ergometers (stationary bikes, elliptical, treadmills, etc.), or performing exercises that do not involve a large joint impact or excessive damage to lower extremities [51]. Training sessions could be made in family health centers, community centers or clinics that have the previously described conditions. Finally, with regard to patients, it is important to note that they may present various complications or pathologies associated with type 2 diabetes mellitus [24]. For example, in the randomized controlled clinical trial analyzed, patients with microvascular complications (retinopathy, nephropathy and / or neuropathy) and cardiovascular diseases were not considered. Therefore, the results of this study cannot be extrapolated to the entire population of patients with type 2 diabetes mellitus.
The most important aspect is that there is a continuous evaluation and monitoring of patients who are doing the exercise program, using parameters such as heart rate reserve, scales of perceived exertion (e.g. the Borg scale), ventilatory rate, among others. It should be emphasized that before starting any training program, patients must undergo a medical examination, followed by an assessment of their physical condition, apart from a period of learning the technical execution of the exercises to be used during training to ensure a progressive intervention and avoid injury [52].
Conclusion
In adult (50-70 years) controlled-patients with type 2 diabetes mellitus both moderate-intensity continuous training, and high-intensity interval training appear to be equally effective in normalizing lipid profile markers and increased general fitness. However, high-intensity interval training seems to be more effective in reducing oxidative stress markers. Due to the high risk of bias of the analyzed trial, more and qualitatively better randomized clinical trials are required in order to confirm or contrast these findings.
Notes
Conflicts of interests
The authors have completed the conflict of interests declaration form from the ICMJE (translated into Spanish by Medwave), and declare not having any conflict of interests with the matter dealt herein. Forms can be requested from the responsible author or the editors.
From the editor
This article was originally submitted in Spanish and was translated into English by the authors. The Journal has not copyedited this version.
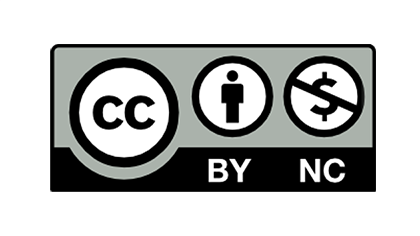