Clinical reviews
← vista completaPublished on December 13, 2023 | http://doi.org/10.5867/medwave.2023.11.2719
Community-acquired pneumonia: Epidemiology, diagnosis, prognostic severity scales, and new therapeutic options
Neumonía adquirida en la comunidad: epidemiología, diagnóstico, escalas pronósticas de gravedad y nuevas opciones terapéuticas
Abstract
This narrative review article explores the current scientific knowledge on the definition, epidemiology, diagnostic criteria, microbiology, treatment, and prevention of severe community-acquired pneumonia (SCAP) in immunocompetent adults. At present, despite major scientific advances in diagnostic evaluation, clinical management, antimicrobial therapy, and prevention, severe community-acquired pneumonia remains a major cause of morbidity and mortality, as well as having a major economic impact in terms of increased healthcare expenditure worldwide. This pathology is considered one of the leading causes of sepsis/septic shock, with an extremely high overall mortality rate, which justifies all the effort in early diagnosis, proper management, and prompt initiation of antimicrobial therapy. Including biomarkers (isolated or in combination) associated with applying diagnostic criteria and prognostic severity scales in clinical practice helps identify patients with severe community-acquired pneumonia, defines immediate admission to the intensive care unit, and, thus, minimizes the adverse outcomes of this serious pathology.
Main messages
- Lower airway infection is one of the leading causes of morbidity worldwide.
- The prognostic severity scales most commonly used in clinical practice to evaluate community-acquired pneumonia are the Pneumonia Severity Index and the CURB-65, both of which perform well in predicting 30-day mortality.
- Early identification of the pathogen causing severe community-acquired pneumonia is an important step in etiological diagnosis since it allows targeting antimicrobial therapy, leading to better clinical outcomes. However, traditional methods used in microbiological diagnosis can only identify pathogens in 30 to 40% of cases of community-acquired pneumonia.
- New clinical trials involving patients with severe community-acquired pneumonia are expected to conduct superiority analyses of long-term adverse events rather than non-inferiority studies of new antibiotics.
Introduction
Lower airway infection is one of the leading causes of morbidity worldwide. Community-acquired pneumonia is the second most frequent cause of hospital admission [1,2]. The incidence of patients requiring intensive care for severe community-acquired pneumonia has been increasing worldwide, mainly in the elderly population (older than 65 years), patients with chronic diseases (diabetes mellitus, dementia, chronic heart failure, ischemic cardiomyopathy), and individuals with some degree of immunosuppression [3].
Jain et al., in 2015, through a large population-based study in which hospitalized patients with a diagnosis of community-acquired pneumonia were followed up, estimated that 21% of these patients were admitted to the intensive care unit and 26% required invasive ventilatory support [4]. In-hospital mortality from severe community-acquired pneumonia is high, ranging from 25% to more than 50% [5,6]. Delayed admission to the intensive care unit is presented as one of the worst prognostic factors and higher in-hospital mortality [7]. Over the years, some prognostic severity scales have been developed and validated in the clinical evaluation of community-acquired pneumonia to identify patients who should be hospitalized and immediately admitted to intensive care units and receive appropriate antimicrobial treatment [8]. Although there is no global consensus on the definition of severe community-acquired pneumonia, the currently accepted criteria are based on international clinical practice guidelines. Those of the Infectious Diseases Society of America/American Thoracic Society (IDSA/ATS), published in 2007 [9] and updated by the same societies in 2019 [10], will be used in this review. The microbial etiology of severe community-acquired pneumonia is not always clear in clinical practice, and different studies show variable results. Streptococcus pneumoniae is the most frequent pathogen associated with community-acquired bacterial pneumonia. The implementation of the polymerase chain reaction (PCR) technique has made it possible to identify the role of respiratory viruses in the etiology of community-acquired pneumonia, particularly influenza virus and rhinovirus [4] and, more recently, the SARS-CoV-2 (COVID-19) pandemic as a cause of severe community-acquired pneumonia [11]. An increased incidence of community-acquired pneumonia caused by a group of antimicrobial multidrug-resistant bacteria, and recently named with the acronym PES (Pseudomonas aeruginosa, broad-spectrum β-lactamase-producing Enterobacteriaceae and methicillin-resistant Staphylococcus aureus), has been observed in patients with certain risk factors [12].
This narrative review explores current knowledge related to the definition, epidemiology, diagnostic criteria, microbial etiology, treatment, and prevention of severe community-acquired pneumonia in immunocompetent adults. Its purpose is to highlight the importance of early diagnosis and appropriate treatment of severe community-acquired pneumonia and improve this condition’s clinical outcomes.
Methods
A literature review was conducted by searching the MEDLINE/PubMed database from 2017 to 2022, using the following terms: pneumonia, severe community-acquired pneumonia, and community-acquired pneumonia. Priority was given to publications of international guidelines, narrative review articles, systematic reviews, and meta-analyses in English. Article abstracts had to contain information on the following parameters: epidemiology, risk factors, clinical manifestations, diagnostic criteria, prognostic severity scales, prevention, and treatment. Only articles with access to the full text were selected. A search was also performed in the UpToDate® database using the term "community-acquired pneumonia" (Figure 1).
Article identification and selection.
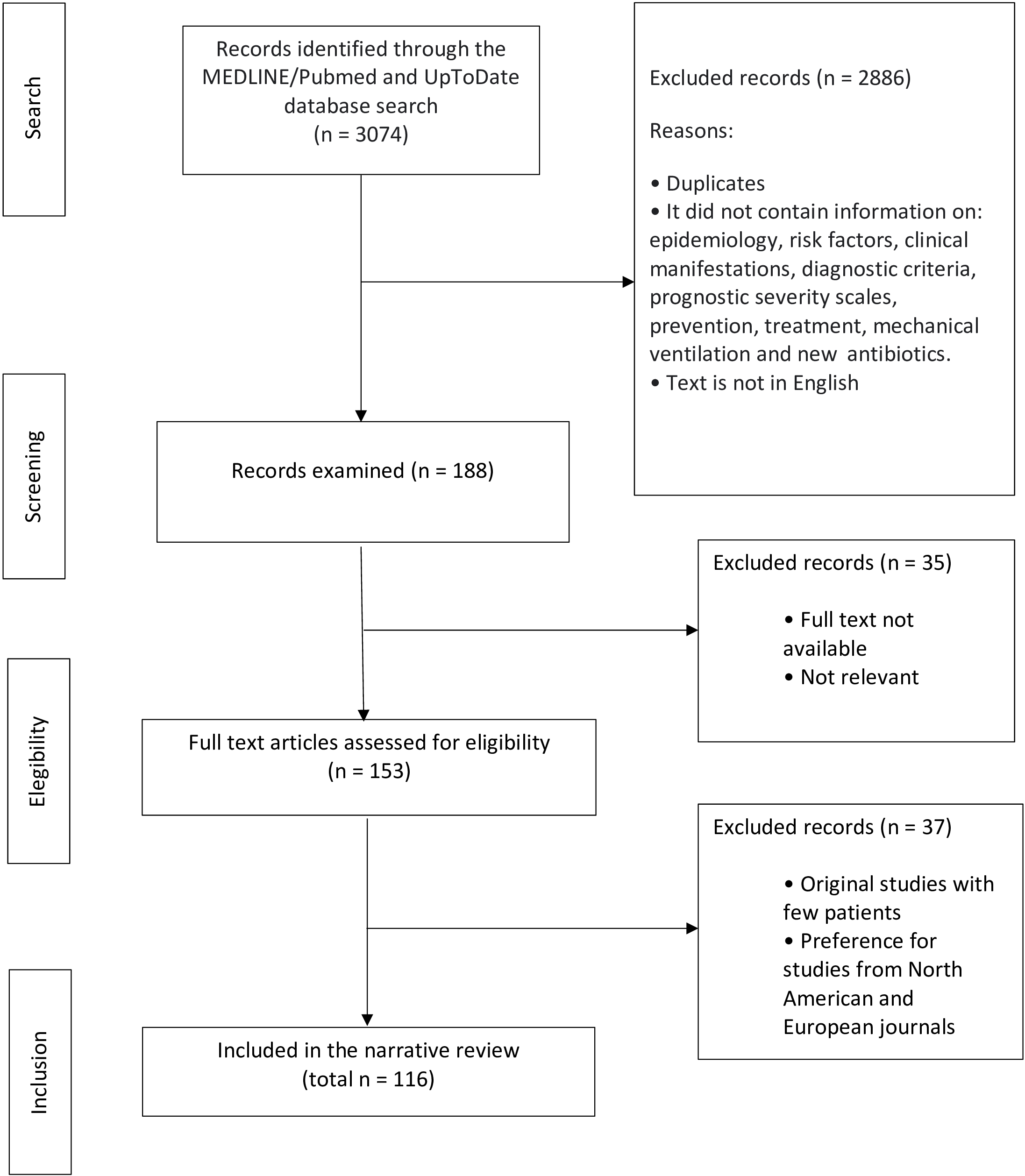
Definition and epidemiology
Community-acquired pneumonia is an acute infection of alveolar units of the lung parenchyma, with onset in the community or within the first 48 hours of hospitalization [13]. It can present with mild forms, characterized by fever and productive cough, or severe forms. Severe community-acquired pneumonia is often a multisystem disease that can progress to multiorgan failure. According to the Infectious Diseases Society of America and American Thoracic Society (IDSA/ATS) criteria, published in 2007 [9], severe community-acquired pneumonia requires the presence of one or more major criteria (need for invasive mechanical ventilation or septic shock requiring vasopressors) or at least three of nine minor criteria: respiratory frequency greater than or equal to 30 breaths per minute, arterial oxygen pressure/inspired oxygen fraction ratio less than 250 millimeters of mercury, multipolar pulmonary infiltrate, confusion/disorientation, blood urea nitrogen greater than or equal to 20 micrograms per deciliter, leukocytes less than 4000 cells per cubic millimeter, platelets less than 100 000 cells per cubic millimeter, body temperature less than 36 degrees Celsius, arterial hypotension requiring fluid resuscitation.
The incidence of hospitalization for community-acquired pneumonia varies considerably depending on geographic location, methodology of the epidemiological study, case definition used, and characteristics of the studied population. Recently, two studies estimated the incidence of hospitalization for community-acquired pneumonia in the United States [4,14]. Griffon et al. identified an annual incidence of hospitalizations for community-acquired pneumonia, per 1000 adults, of 1.2 for individuals aged 65 to 74 years; 2.39 for those aged 75 to 84 years; and 4.39 for individuals aged 85 years or older in the period from 2007 to 2009 [14] They also observed a higher incidence of severe disease at older ages. Similarly, in a Portuguese study conducted from 2000 to 2009, Froes et al. reported a hospitalization rate for community-acquired pneumonia of 3.61 per 1000 adult individuals, with a significant increase of 13.3 per 1000 in the group aged 65 years or older. The authors also observed that from 2000 to 2009, the annual hospitalization rate for community-acquired pneumonia per 1000 individuals increased by 28.2% [15].
In Brazil, community-acquired pneumonia is the leading cause of hospitalization in the Unified Health System. In 2017, 598 688 community-acquired pneumonia hospitalizations took place [16].
Risk factors associated with increased mortality from community-acquired pneumonia include advanced age (over 65 years), presence of chronic diseases (chronic obstructive pulmonary disease, bronchiectasis, asthma, chronic heart disease, stroke, diabetes mellitus), sepsis, septic shock, inadequate antibiotic therapy, need for mechanical ventilation and hypoalbuminemia [9].
For their part, Restrepo et al. observed that delay in the initiation of proper antimicrobials or delay in admission to the intensive care unit were associated with adverse prognoses in the evolution of patients hospitalized for severe community-acquired pneumonia [17].
Likewise, the presence of active smoking significantly increases the risk of developing community-acquired pneumonia, mainly when linked to immunodeficiency [18].
Prognostic severity scales
Early identification of patients with severe community-acquired pneumonia is considered a fundamental step in the initial evaluation of patients and very necessary for the definition of the swiftest and optimal clinical treatment, in addition to avoiding delays in the transfer of the patient to the intensive care unit and, therefore, minimizing unfavorable outcomes related to the severity of the disease [19,20].
Multiple prognostic severity scales have been validated and are used in the initial clinical evaluation to help decide whether to treat community-acquired pneumonia in the outpatient or inpatient setting. However, most still fail to predict the need for admission to the intensive care unit [21]. Identifying the most suitable setting for clinical management of the patient with severe community-acquired pneumonia is an important variable to be assessed through prognostic severity scales. Clinical studies identified that the length of hospitalization and in-hospital mortality was higher among patients with severe community-acquired pneumonia who inadvertently initiated treatment in a non-acute inpatient unit and were subsequently transferred to the intensive care unit, compared with those who were appropriately transferred from the emergency department to the intensive care unit [17,22].
The most commonly used prognostic severity scales in clinical practice for the evaluation of community-acquired pneumonia are the Pneumonia Severity Index (PSI) and the CURB-65 (acronym for confusion: score less than eight on the abbreviated mental test; urea: greater than 43 milligrams per deciliter or blood urea nitrogen greater than 7 millimol; respiration: rate greater than 30 breaths per minute; blood pressure: systolic less than 90 millimeters of mercury or diastolic less than or equal to 60 millimeters of mercury; and age greater than or equal to 65 years). Both tools work well for predicting 30-day mortality but are of little use in identifying severe community-acquired pneumonia requiring intensive care unit admission [23]. On this, Torres et al. identified that predictors of mortality in community-acquired pneumonia are strongly influenced by patient age and very little by the presence of respiratory failure and other organ dysfunction, which often lead to the need for intensive care [22].
Other prognostic scales have been proposed, such as IDSA/ATS 2007, simplified IDSA/ATS minor criteria, SMART-COP (systolic blood pressure less than 90 millimeters of mercury; multilobe involvement; albumin less than 3.5 grams per deciliter; age-adjusted respiratory rate; tachycardia less than or equal to 125; confusion; age-adjusted oxygenation; pH less than 7.35), SCAP (Severe Community-Acquired Pneumonia), and more aimed at identifying the existence of organ dysfunction in patients with community-acquired pneumonia. The IDSA/ATS 2007 clinical and laboratory criteria are the most pragmatic and robust for identifying patients with severe community-acquired pneumonia requiring admission to the intensive care unit [22] (Table 1).
Recently, it has been shown that the diagnostic criteria for Sepsis-3, represented by the q-SOFA (respiratory rate greater than or equal to 22 respiratory forays per minute, altered mental status, and systolic blood pressure less than or equal to 100 millimeters of mercury), can also aid in the identification of patients with severe community-acquired pneumonia seen in the emergency department. However, the use of disease-specific instruments (Pneumonia Severity Index and CURB-65) has shown better discriminatory power in predicting the risk of death [24].
Microbiological diagnosis
Early microbiological identification in severe community-acquired pneumonia is an important step toward etiological diagnosis, allows de-escalating antimicrobial therapy, and results in better clinical outcomes. However, traditional methods used in microbiological diagnosis can only identify pathogens in 30 to 40% of cases of community-acquired pneumonia [4,25]. Cillóniz et al. concluded that blood cultures have low diagnostic sensitivity, with an estimated bacterial detection rate between 5% and 15%. They have an important clinical value when positive, as they help direct antimicrobial therapy. The possibility of a shorter time for blood culture results (less than 10 hours) is considered an independent risk factor for the need for invasive mechanical ventilation, longer hospital stays, higher in-hospital mortality, and higher 30-day mortality [26]. Despite the fact that PCR-based molecular diagnostic techniques have improved diagnostic rates for some viruses and bacteria most commonly related to the etiology of community-acquired pneumonia [27,28], the diagnostic value of these techniques is still restricted to a low number of microbial agents [29]. Recently, the next-generation metagenomic sequencing technique has become a powerful and promising diagnostic tool in identifying microorganisms responsible for various infectious diseases, the results of which are not affected by the prior use of antibiotic therapy. Scientific studies have been published on the use of next-generation metagenomic sequencing in material obtained from bronchoalveolar lavage used to identify the microorganisms responsible for severe community-acquired pneumonia [30,31]. Implementing these new diagnostic techniques in clinical practice is partially difficult, partly due to their high cost and the lack of standardization of these tests. Another factor to consider is the high sensitivity of these techniques, which could make it difficult to differentiate between colonization and infection [21].
In the 2019 publication, the IDSA/ATS, through the guideline for the diagnosis and treatment of community-acquired pneumonia in adults, suggested that etiologic diagnosis should be obtained by Gram stain techniques, lower respiratory tract cultures, blood cultures, presence of Legionella and pneumococcal urinary antigens. It also suggests viral testing for influenza and coronavirus in adult patients requiring hospitalization classified with severe community-acquired pneumonia [32] or with risk factors for infection by methicillin-resistant Staphylococcus aureus (MRSA) or Pseudomonas aeruginosa (Table 2).
Streptococcus pneumoniae remains the most common bacterial pathogen in severe community-acquired pneumonia, regardless of age or comorbidities [9]. Although an increased incidence of antimicrobial-resistant S. pneumoniae strains has been observed, Gattarello et al. reported a decreased in-hospital mortality in patients admitted to the intensive care unit with a diagnosis of severe community-acquired pneumococcal pneumonia, possibly due to early initiation of antimicrobials and combined therapy [33]. On the other hand, in a retrospective cohort study of patients diagnosed with severe community-acquired pneumonia, Valles et al. identified S. pneumoniae as the most frequent pathogen, with a total incidence of 41.6% and over 80% when combining all causes of bacteremia [34]. Other pathogens involved in the etiology of severe community-acquired pneumonia are viruses (influenza and its serotypes, respiratory syncytial virus, SARS-CoV-2, MERS-CoV, human rhinovirus), atypical bacteria (Legionella pneumophila, Mycoplasma pneumoniae), Haemophilus influenzae, Staphylococcus aureus (including methicillin-resistant S. aureus), gram-negative enterobacteria (Pseudomonas aeruginosa, Acinetobacter baumannii, Klebsiella pneumoniae) and more rarely, anaerobic bacteria.
Novel molecular diagnostic techniques have made it possible to detect viral particles in airway samples from patients with pneumonia and to identify an increased incidence of viral etiology in severe community-acquired pneumonia [4,35,36]. More recently, the SARS-CoV-2 virus originating in Wuhan, China, in 2019 (COVID-19) has been identified as a cause of severe community-acquired pneumonia and has rapidly generated a global pandemic because of its high transmission rates. Even though viral respiratory infections are considered a leading cause of severe community-acquired pneumonia, Qhah et al. observed that among patients with bacterial coinfection, there was an increased risk of in-hospital mortality [37]. Several viral mechanisms, such as bronchial epithelial wall disruption, up-regulation of cell adhesion proteins, or immune system dysregulation, may facilitate concomitant bacterial infection [38]. This synergism may explain the worse prognosis of patients with severe community-acquired pneumonia caused by viral-bacterial coinfection, mainly between influenza virus and Streptococcus pneumoniae [39]. The atypical pathogens most frequently associated with severe community-acquired pneumonia are Legionella pneumophila and Mycoplasma pneumoniae; the prevalence of Chlamydia pneumoniae as a cause of severe community-acquired pneumonia is less than 1%, according to recent studies [40,41]. At the same time, Mycoplasma pneumoniae produces, in most cases, a mild and self-limited disease. In contrast, Legionella pneumophila can cause severe community-acquired pneumonia [42,43]. For an atypical pathogen to be identified as the cause of community-acquired pneumonia, the patient must have a positive culture, a positive polymerase chain reaction or urinary antigen detection for Legionella pneumophila or even a positive oropharyngeal swab for Mycoplasma pneumoniae, in the absence of another bacterial agent [44].
Pseudomonas aeruginosa, broad-spectrum β-lactamase-producing enterobacteria, and methicillin-resistant Staphylococcus aureus represent another important group of severe community-acquired pneumonia-causing pathogens. This bacterial group accounts for approximately 6% of severe community-acquired pneumonia hospitalizations [12]. Risk factors for infection with β-lactamase-producing enterobacteria and methicillin-resistant S. aureus include previous use of parenteral antimicrobials in the last 180 days, low functionality (Barthel functional index less than 50), previous hospitalization greater than 48 hours in the last 90 days, need for hemodialysis, and immunosuppression [45].
Treatment
According to the IDSA/ATS guidelines for the clinical management of community-acquired pneumonia in immunocompetent adult individuals, published in 2019, the antimicrobial therapy of choice will depend on the treatment setting, i.e., in-hospital or outpatient [32]. Empiric antimicrobial therapy should be initiated as soon as the diagnosis of severe community-acquired pneumonia is made and is based on the following criteria: analysis of local microbiology, most likely pathogen, presence of comorbidities and/or existence of risk factors for antimicrobial-resistant pathogens [46].
Once severe community-acquired pneumonia is diagnosed and culture specimens are collected, antimicrobials should be initiated immediately with the goal of reducing disease progression and improving prognosis with decreased in-hospital mortality. The American Thoracic Society guidelines for the treatment of community-acquired pneumonia in adults recommend that antibiotics be administered within four hours of diagnosis [47]. It is worth noting that, in the 2019 publication, the IDSA/ATS has advised discontinuing the term "healthcare-associated pneumonia", used in the 2007 publication [9]. This change of nomination aims to avoid the excessive use of empirical therapy based on broad-spectrum antimicrobials since clinical studies did not show an increased incidence of antibiotic-resistant bacteria in this population [48,49]. In relation to the pharmacokinetic and pharmacodynamic characteristics of antimicrobial therapy in critically ill patients, the organic concentrations of antibiotics show oscillations in both plasma and extracellular fluid, especially in concomitant clinical conditions such as acute kidney injury and hyperdynamic circulatory state present in septic patients, which may compromise the efficacy of the drug.
When deciding the most appropriate antimicrobial for severe community-acquired pneumonia based on polymerase chain reaction therapy, all patients should be evaluated for the presence of risk factors for antibiotic-resistant bacteria. Currently, the most important individual risk factors for respiratory infection with methicillin-resistant Staphylococcus aureus and Pseudomonas aeruginosa are previous culture isolation of these microorganisms, especially from the respiratory tract, previous hospitalization for more than 48 hours in the past 90 days, and history of intravenous antibiotic treatment [50,51,52]. To date, no scoring system with reliable post-test probability exists in clinical practice to identify patients at risk of respiratory infection by multidrug-resistant bacteria [32].
The IDSA/ATS guidelines [32] recommend that patients with severe community-acquired pneumonia admitted to the intensive care unit and without risk factors for antimicrobial-resistant bacteria be treated with dual therapy, i.e., beta-lactam associated with macrolide or respiratory quinolone (levofloxacin or moxifloxacin). Studies show that the therapeutic association between beta-lactam and a macrolide has a benefit in reducing mortality [53,54,55,56]. In this line, three theories can explain this fact: better coverage for atypical pathogens, including Legionella; suppression of endotoxin production (such as pneumolysin) by Streptococcus pneumoniae [57]; and immunomodulatory effect in the patient’s organism.
On the other hand, in patients with severe community-acquired pneumonia and with risk factors for antibiotic-resistant bacterial infection, the IDSA/ATS 2019 guidelines [32], recommend empirical therapy according to the suspected microorganism. If it is methicillin-resistant Staphylococcus aureus, vancomycin (15 milligrams per kilogram every 12 hours, adjusted according to serum level or concentration measurements according to pharmacokinetic and pharmacodynamic parameters) or linezolid (600 milligrams every 12 hours). If Pseudomonas aeruginosa: piperacillin/tazobactam (4.5 grams every 6 hours), cefepime (2 grams every 8 hours), ceftazidime (2 grams every 8 hours) or meropenem (1 gram every 8 hours).
Viral etiology is recognized as a common cause of severe community-acquired pneumonia, and viral and bacterial coinfection is observed in many patients [21]. The Infectious Diseases Society of America/American Thoracic Society (IDSA/ATS) guidelines recommend the use of anti-influenza treatment (oseltamivir) in all patients with documented severe influenza infection, regardless of the duration of symptoms [32]; although it is recognized that the greatest antiviral benefit comes within 48 hours of symptom onset or hospitalization [58]. Additionally, clinical studies demonstrate that it is beneficial to initiate antiviral treatment up to four to five days after symptom onset [59,60]. Also, due to the high incidence of viral and bacterial coinfection, the association of antibiotic therapy is recommended, especially to cover Streptococcus pneumoniae and Staphylococcus aureus. However, antibiotic de-escalation should be performed in case there is no clinical or laboratory evidence of bacterial coinfection and the patient is clinically stable after 48 to 72 hours of treatment [32].
Additionally, in patients with COVID-19, bacterial pneumonia is frequently observed [61,62]. Most patients with pulmonary infiltration on imaging tests suggest that they should receive antibiotic therapy, which should be discontinued based on clinical evaluation and serum levels of biomarkers such as procalcitonin [63,64]. Also in hospitalized patients with COVID-19, the use of the antiviral drug remdesivir showed some benefit, particularly when started early in the course of the disease [65]. The ACT-1 study [66] has shown that remdesevir was superior to placebo in reducing recovery time in hospitalized adults, while the SOLIDARITY study [67] showed no benefit in reducing mortality. Currently, remdesevir is recommended in patients with severe COVID-19 in need of oxygen therapy, excluding mechanical ventilation and extracorporeal respiratory support [68].
Corticosteroids
Regardless of the underlying pathogen, lower airway infection is associated with a significant local, and in some cases systemic, cytokine-mediated inflammatory response, resulting in altered capillary permeability of the alveolar membrane and leaking of inflammatory contents into the alveoli, also being the determinant mechanism of the severity of respiratory disease [69]. Corticosteroids suppress the expression of genes related to the production of proinflammatory proteins, which explains their anti-inflammatory effect [70]. Because of their immunomodulatory effects, corticosteroids are frequently used as adjunctive therapy in treating severe community-acquired pneumonia [71]. However, their efficacy and safety remain questionable [72].
In recent years, several systematic reviews and meta-analyses have been published exploring the efficacy of corticosteroid therapy in treating severe community-acquired pneumonia. In 2017, Cochrane published a meta-analysis that included 17 randomized clinical trials with 2264 patients, which evidenced a reduction in mortality and morbidity among adults diagnosed with severe community-acquired pneumonia [73]. More recently, a meta-analysis revealed some differences in outcomes, depending on the type of corticosteroid used, with prednisolone and methylprednisolone therapy significantly reducing patient mortality, whereas this was not observed with the use of hydrocortisone. The authors also observed that the length of stay in the intensive care unit was significantly lower for the group of patients using corticosteroids compared to the control group, also showing a tendency of lower need for mechanical ventilation in patients in the intervention group [72]. Already Meduri et al., through the ESCAPe study (double-blind, randomized, placebo-controlled clinical trial), studied 584 patients hospitalized in the intensive care unit for severe community-acquired pneumonia, whose intervention group received methylprednisolone for 20 days: 40 milligrams per day for 7 days, 20 milligrams per day for the next 7 days, 12 milligrams per day for 3 days, and finally 4 milligrams per day for the last three days, observed that there was no significant reduction in 60-day mortality compared with the control group [74].
Recently, PF Dequien et al., through the CAPE COD Trial (multicenter, double-blind, randomized, placebo-controlled trial), in which 800 patients with severe community-acquired pneumonia hospitalized in the intensive care unit were evaluated, showed that the use of hydrocortisone at a dose of 200 milligrams per day, compared with placebo, decreased mortality at 28 days, and was associated with lower need for vasopressors and mechanical ventilation [75].
Currently, guidelines recommend against the use of corticosteroids in the treatment of severe community-acquired pneumonia due to the risk of influenza infection. However, they mention that it may be important in the treatment of severe bacterial pneumonia, especially in cases of septic shock or community-acquired pneumonia by pneumococcus, and in pneumococcal pneumonia associated with meningitis [32].
On the other hand, several clinical studies suggest that the use of corticosteroids as adjunctive therapy in the treatment of patients with severe influenza pneumonia may be associated with higher mortality rates, as well as with an increase in the number of days on mechanical ventilation, the length of hospitalization and an increased rate of opportunistic infections, possibly as a result of concomitant immunosuppression [76,77,78].
In contrast to severe community-acquired influenza pneumonia, patients with severe COVID-19, i.e., those who progress with respiratory failure and need of non-invasive oxygen therapy or invasive mechanical ventilation, have better survival at 28 days when treated with corticosteroids [79]. Similar findings were found in patients with moderate or severe acute respiratory distress syndrome by COVID-19 requiring invasive mechanical ventilation, in whom the use of corticosteroids at higher doses was associated with reduced mortality and need for mechanical ventilation at 28 days [75,80]. This benefit of corticosteroid use in COVID-19 was subsequently reinforced by a meta-analysis that included seven randomized controlled studies involving a total of 1703 critically ill patients with COVID-19 from 12 different countries, in whom a decrease in all-cause mortality at 28 days was evident [81].
Biomarkers
Biomarkers are objectively measured substances that can be used as predictors of a physiological or pathological process, of a response to a therapeutic intervention, or of a disease’s progression [82]. Biomarkers of infection should possess characteristics that facilitate diagnosis, prognosis, and clinical follow-up [83]. In pneumonia, several studies have evaluated the role of biomarkers in diagnosis, etiology, risk stratification, and disease severity, as well as initiation, duration, and discontinuation of antimicrobial therapy [84]. C-reactive protein and procalcitonin are the most studied inflammatory biomarkers used in clinical practice [85].
C-reactive protein is an important acute-phase protein produced by macrophages in response to any type of inflammation, including bacterial and viral infections. It is detected in the blood between four and six hours after the initial stress, reaching maximum concentrations between 36 and 48 hours. Despite this, C-reactive protein has a low specificity in diagnosing pneumonia, being elevated in other inflammatory conditions, such as neoplasia and autoimmune diseases, and non-infectious acute inflammatory processes, such as trauma [86].
Procalcitonin is a peptide precursor of the calcitonin hormone, produced in the thyroid gland and elevates during infections and inflammatory diseases, primarily as an acute phase reactant produced by the liver. In healthy individuals, the concentration of procalcitonin is extremely low (less than 0.1 nanograms per milliliter). However, its levels are elevated during bacterial infections [86]. In hospitalized patients with community-acquired pneumonia, procalcitonin has been studied with the aim of using it as a tool that can help reduce the duration of antimicrobial therapy. Several studies have been using serial measurements of procalcitonin as a parameter for the creation of clinical algorithms to safely determine the discontinuation of antibiotic therapy [87,88,89].
For instance, Salluh et al. proposed an algorithm for initiating antibiotic therapy with a predetermined duration. Subsequently, serial measurements of procalcitonin in combination with the clinical course of the patient with community-acquired pneumonia are used to securely determine the time of antimicrobial discontinuation [90].
New antibiotics
In recent decades, much effort has been put into researching and developing new antimicrobial drugs for treating severe bacterial community-acquired pneumonia (Table 3). However, these new antibiotics are frequently directed to infections by multidrug-resistant bacteria.
Most of the clinical trials were conducted in patients with severe community-acquired pneumonia of bacterial etiology and without severity criteria; patients with severe community-acquired pneumonia requiring intensive care unit hospitalization were excluded [91,92,93,94,95,96,97,98]. Recommendations for the use of these new drugs in the group of patients with severe community-acquired pneumonia are based on clinical studies in which these patients were not adequately represented. Therefore, we hope that new clinical trials conducting superiority rather than non-inferiority analyses of the new antibiotics, including patients with severe community-acquired pneumonia and also evaluating long-term adverse events, will emerge [99].
Despite the fact that Streptococcus pneumoniae is the main pathogen responsible for severe community-acquired pneumonia, in recent decades, there has been growing interest in the role of gram-negative bacteria in this type of pneumonia, especially in patients with chronic lung disease and in those with a history of antimicrobial use [100].
Increased bacterial resistance is a limiting factor in treating pneumonia caused by multidrug-resistant gram-negative bacteria, hindering effective antibiotic therapy and making this condition a global public health concern. Moreover, this increases healthcare costs, hospitalization times, and mortality [101].
For this reason, several new antimicrobials have been developed to increase the therapeutic alternatives against germs that may present different resistance mechanisms to commonly used antimicrobials. One example is ceftobiprole, a fifth-generation cephalosporin with activity against gram-negative bacteria such as Haemophilus influenzae, Moraxella catarrhalis, and Pseudomonas aeruginosa, weak activity against bacteria that produce β-lactamases of the AmpC-type, and no activity against Enterobacteriaceae that produce extended-spectrum β-lactamases, carbapenemase and metallo-β-lactamases. In addition, it does not exhibit activity against Burkholderia cepacia and Stenotrophomonas maltophilia. Ceftobiprole also exhibits significant activity against gram-positive germs, such as Streptococcus pneumoniae and methicillin-resistant Staphylococcus aureus [102].
On the other hand, ceftolozane-tazobactam is a combination of the modified cephalosporin ceftolozane with the β-lactamase inhibitor tazobactam. Ceftolozane exhibits stability against different resistance mechanisms of gram-negative bacteria such as AmpC-type β-lactamase producers and efflux pumps, in addition to being the β-lactam with the highest activity against Pseudomonas aeruginosa, including multidrug-resistant strains. The ceftolozane-tazobactam combination shows activity against Enterobacteriaceae, producing extended-spectrum β-lactamases. However, it shows no activity against carbapenemase-producing gram-negatives, including Pseudomonas aeruginosa. This combination also lacks effect against Acinetobacter baumannii and Stenotrophomonas maltophilia [103].
Already, ceftazidime-avibactam is a combination consisting of a third-generation cephalosporin and a non-β-lactam β-lactamase inhibitor. Avibactam protects ceftazidime from hydrolysis caused by different classes of β-lactamases, such as type A (broad-spectrum β-lactamase-producing enterobacteria and carbapenemase-producing Klebsiella pneumoniae), type C (AmpC-type β-lactamase-producing) and type D (OXA-48) β-lactamases. However, it has low activity against β-lactamases of type B, Acinetobacter baumannii, anaerobic gram-negative bacteria, and gram-positive cocci. Ceftazidime-avibactam is also active against carbapenem-resistant Enterobacteriaceae, where 80% of isolated strains have shown susceptibility in vitro. About 90% of isolated Pseudomonas aeruginosa strains show susceptibility to ceftazidime-avibactam, as well as about two-thirds of non-ceftazidime-susceptible strains and three-quarters of carbapenem-resistant P. aeruginosa strains are susceptible to this combination [104,105].
Cefiderocol is a novel modified cephalosporin with a catechol side chain that forms a chelated complex with iron. Cefiderocol inhibits cell wall synthesis by binding to penicillin-binding proteins, thus slowing peptidoglycan synthesis. This new type of cephalosporin maintains its same activity in the presence of β-lactamases type A, B, C, and D. In more than 28 000 strains of gram-negative bacteria isolated, more than 99% of enterobacteria (Escherichia coli, Klebsiella spp, Citrobacter spp, and Serratia spp) were found to be susceptible to cefiderocol. Similarly, in vitro susceptibility was found for Pseudomonas aeruginosa, Burkholderia cepacia, and Stenotrophomonas maltophilia [106,107].
Ventilatory support
The main aspect of non-pharmacological treatment of the patient with severe community-acquired pneumonia is respiratory support, ranging from conventional oxygen therapy, non-invasive mechanical ventilation, and high-flow nasal cannula to invasive mechanical ventilation.
Non-invasive mechanical ventilation and high-flow nasal cannula are two methods of respiratory support that decrease respiratory work. These procedures provide better oxygenation, aiming to avoid intubation and decrease mortality in patients with acute hypoxemic respiratory failure. According to the current literature, the decision between using non-invasive mechanical ventilation or high-flow nasal cannula remains uncertain.
In patients with acute respiratory failure secondary to pneumonia, non-invasive mechanical ventilation with a Helmet could decrease the need for endotracheal intubation compared to conventional oxygen therapy with a venturi mask [108]. A meta-analysis of three randomized clinical trials with 151 patients showed that using non-invasive mechanical ventilation in pneumonia can decrease the need for endotracheal intubation, mortality in intensive care, and hospitalization time in the intensive care unit [109]. In the immunosuppressed group, although non-invasive mechanical ventilation was able to reduce the need for endotracheal intubation in patients with fever, pulmonary infiltrate, and hypoxemic acute respiratory failure [110], early use of this modality of non-invasive ventilatory support that is, with less than 72 hours after the onset of respiratory failure; was unable to decrease mortality at 28 days of follow-up in this same group of patients, compared to conventional oxygen therapy [111].
Even though high-flow nasal cannula oxygen therapy provides high oxygen concentrations at and low positive end-expiratory pressure levels, the main study comparing high-flow nasal cannula oxygen therapy, conventional oxygen therapy, and non-invasive mechanical ventilation showed no difference in the rate of endotracheal intubation at 28 days in patients with hypoxemic acute respiratory failure. It is important to highlight that this same study found a lower mortality at 90 days in the group of patients submitted to oxygen therapy by high-flow nasal cannula [112].
Both high-flow nasal cannula oxygen therapy and non-invasive mechanical ventilation are widely available approaches to respiratory support and are therefore indicated in cases of community-acquired pneumonia with acute hypoxemic respiratory failure instead of conventional oxygen therapy to avoid endotracheal intubation when there is no need for immediate intubation [113]. So far, a comparison between non-invasive mechanical ventilation and high-flow nasal cannula in community-acquired pneumonia is not available in the literature.
Invasive mechanical ventilation may be necessary in patients with severe community-acquired pneumonia. Late endotracheal intubation of patients with severe pneumonia and acute respiratory failure may increase mortality [114]. In cases of community-acquired pneumonia with severe acute respiratory distress syndrome, using protective mechanical ventilation settings, i.e., low tidal volumes (4 to 8 milliliters per kilogram), is strongly recommended [115]. In patients with less severe pneumonia, the protective strategy may decrease the risk of progression to mechanical ventilation-induced lung injury [116].
Conclusions
The morbimortality of severe community-acquired pneumonia is still high, especially among elderly, chronically ill, and immune-compromised patients. Improving preventive measures, such as increased vaccination rates in the adult population and promoting smoking cessation, are very important. Prognostic severity scales can help diagnose the most severe cases of community-acquired pneumonia, thus helping to define the treatment regimen to be followed, whether in the outpatient, inpatient, or intensive care unit. Early initiation of antimicrobial treatment, especially in severe cases, is essential to improve survival and reduce the risk of complications related to community-acquired pneumonia. Although several studies show a benefit in the use of corticosteroids in treating severe pneumonia, their use remains controversial, especially in mild cases and in some viral pneumonia. The increased incidence of multidrug-resistant pathogens, as well as the aging of the population and higher prevalence of comorbidities, are challenges that are increasingly present in clinical practice. New studies are needed to evaluate the efficacy and efficiency of new antimicrobials in community-acquired pneumonia caused by multidrug-resistant bacteria.
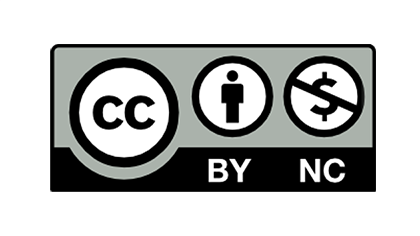